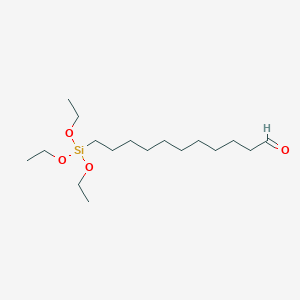
11-Triethoxysilylundecanal
Overview
Description
Triethoxysilylundecanal is a chemical compound with the molecular formula C17H36O4Si and a molecular weight of 332.56 g/mol . It is an aldehyde functional trialkoxy silane, which means it contains both an aldehyde group and three ethoxy groups attached to a silicon atom. This compound is known for its ability to form durable bonds between organic and inorganic materials, making it a valuable silane coupling agent .
Safety and Hazards
Triethoxysilylundecanal may cause skin irritation and serious eye irritation . It may also cause respiratory irritation . Overexposure to ethanol by skin absorption, inhalation, or ingestion may have a narcotic effect (headache, nausea, drowsiness) . This product contains ethanol which is classified as a carcinogen by IARC in alcoholic beverages .
Mechanism of Action
Target of Action
Triethoxysilylundecanal is a type of organoethoxysilane . It is primarily used as a chemical intermediate . The primary targets of Triethoxysilylundecanal are organic and inorganic materials. It forms a durable bond between these materials to generate desired heterogeneous environments or to incorporate the bulk properties of different phases into a uniform composite structure .
Mode of Action
Triethoxysilylundecanal interacts with its targets by forming a durable bond between organic and inorganic materials . This interaction results in the generation of heterogeneous environments or the incorporation of the bulk properties of different phases into a uniform composite structure .
Biochemical Pathways
As an organoethoxysilane, it is known to participate in silane coupling reactions . These reactions can lead to the formation of durable bonds between organic and inorganic materials, affecting the properties of the resulting composite materials .
Result of Action
The primary result of Triethoxysilylundecanal’s action is the formation of a durable bond between organic and inorganic materials . This leads to the generation of heterogeneous environments or the incorporation of the bulk properties of different phases into a uniform composite structure .
Action Environment
The action of Triethoxysilylundecanal can be influenced by environmental factors. For instance, additional ethanol may be formed by reaction with moisture and water . This could potentially affect the compound’s action, efficacy, and stability .
Biochemical Analysis
Biochemical Properties
Triethoxysilylundecanal plays a significant role in biochemical reactions. It interacts with various enzymes, proteins, and other biomolecules. The organofunctional group of Triethoxysilylundecanal alters the wetting or adhesion characteristics of the substrate, utilizes the substrate to catalyze chemical transformations at the heterogeneous interface, orders the interfacial region, or modifies its partition characteristics .
Molecular Mechanism
The molecular mechanism of Triethoxysilylundecanal involves the formation of stable condensation products with siliceous surfaces and other oxides such as those of aluminum, zirconium, tin, titanium, and nickel . It exerts its effects at the molecular level, including binding interactions with biomolecules and potential enzyme inhibition or activation .
Preparation Methods
Triethoxysilylundecanal can be synthesized through various methods. One common approach involves the reaction of undecanal with triethoxysilane in the presence of a catalyst. The reaction typically occurs under mild conditions, such as room temperature, and may require a solvent like ethanol to facilitate the process . Industrial production methods often involve the use of vapor-phase techniques to functionalize surfaces with triethoxysilylundecanal .
Chemical Reactions Analysis
Triethoxysilylundecanal undergoes several types of chemical reactions, including:
Oxidation: The aldehyde group can be oxidized to form a carboxylic acid.
Reduction: The aldehyde group can be reduced to form a primary alcohol.
Substitution: The ethoxy groups can be hydrolyzed to form silanol groups, which can further condense to form siloxane bonds.
Common reagents used in these reactions include oxidizing agents like potassium permanganate for oxidation, reducing agents like sodium borohydride for reduction, and water or acids for hydrolysis . The major products formed from these reactions include carboxylic acids, primary alcohols, and siloxane polymers .
Scientific Research Applications
Triethoxysilylundecanal has a wide range of scientific research applications:
Comparison with Similar Compounds
Triethoxysilylundecanal is unique due to its long alkyl chain, which provides greater stability for coupled proteins compared to shorter alkyl homologs . Similar compounds include:
Triethoxysilylbutyraldehyde: A shorter chain homolog with similar coupling properties but less stability.
Triethoxysilylpropionaldehyde: Another shorter chain homolog with similar reactivity but reduced stability.
Properties
IUPAC Name |
11-triethoxysilylundecanal | |
---|---|---|
Source | PubChem | |
URL | https://pubchem.ncbi.nlm.nih.gov | |
Description | Data deposited in or computed by PubChem | |
InChI |
InChI=1S/C17H36O4Si/c1-4-19-22(20-5-2,21-6-3)17-15-13-11-9-7-8-10-12-14-16-18/h16H,4-15,17H2,1-3H3 | |
Source | PubChem | |
URL | https://pubchem.ncbi.nlm.nih.gov | |
Description | Data deposited in or computed by PubChem | |
InChI Key |
SJJMMZVIBLQHLI-UHFFFAOYSA-N | |
Source | PubChem | |
URL | https://pubchem.ncbi.nlm.nih.gov | |
Description | Data deposited in or computed by PubChem | |
Canonical SMILES |
CCO[Si](CCCCCCCCCCC=O)(OCC)OCC | |
Source | PubChem | |
URL | https://pubchem.ncbi.nlm.nih.gov | |
Description | Data deposited in or computed by PubChem | |
Molecular Formula |
C17H36O4Si | |
Source | PubChem | |
URL | https://pubchem.ncbi.nlm.nih.gov | |
Description | Data deposited in or computed by PubChem | |
DSSTOX Substance ID |
DTXSID40467911 | |
Record name | 11-triethoxysilylundecanal | |
Source | EPA DSSTox | |
URL | https://comptox.epa.gov/dashboard/DTXSID40467911 | |
Description | DSSTox provides a high quality public chemistry resource for supporting improved predictive toxicology. | |
Molecular Weight |
332.5 g/mol | |
Source | PubChem | |
URL | https://pubchem.ncbi.nlm.nih.gov | |
Description | Data deposited in or computed by PubChem | |
CAS No. |
116047-42-8 | |
Record name | 11-(Triethoxysilyl)undecanal | |
Source | CAS Common Chemistry | |
URL | https://commonchemistry.cas.org/detail?cas_rn=116047-42-8 | |
Description | CAS Common Chemistry is an open community resource for accessing chemical information. Nearly 500,000 chemical substances from CAS REGISTRY cover areas of community interest, including common and frequently regulated chemicals, and those relevant to high school and undergraduate chemistry classes. This chemical information, curated by our expert scientists, is provided in alignment with our mission as a division of the American Chemical Society. | |
Explanation | The data from CAS Common Chemistry is provided under a CC-BY-NC 4.0 license, unless otherwise stated. | |
Record name | 11-triethoxysilylundecanal | |
Source | EPA DSSTox | |
URL | https://comptox.epa.gov/dashboard/DTXSID40467911 | |
Description | DSSTox provides a high quality public chemistry resource for supporting improved predictive toxicology. | |
Synthesis routes and methods
Procedure details
Q1: How does Triethoxysilylundecanal interact with surfaces to create functional monolayers?
A: Triethoxysilylundecanal (TESUD) forms self-assembled monolayers (SAMs) on oxide surfaces like silicon dioxide (SiO2). This interaction involves the hydrolysis of the triethoxysilane groups in TESUD with surface hydroxyl groups, followed by condensation reactions that lead to the formation of a stable Si-O-Si network. [, , , ] This process results in a monolayer of TESUD molecules with their aldehyde (-CHO) groups exposed at the surface, which can be further functionalized. [, , , ]
Q2: Can the surface properties of Triethoxysilylundecanal SAMs be modified?
A: Yes, the terminal aldehyde groups of TESUD SAMs offer a versatile platform for further modifications. For instance, they can be photochemically oxidized to carboxylic acid (-COOH) groups using vacuum UV light. [, , ] This transformation allows for the creation of surfaces with distinct chemical reactivity, enabling the attachment of biomolecules like proteins for applications such as biosensing. [, ]
Q3: What are the advantages of using Triethoxysilylundecanal in biosensing applications?
A: TESUD offers several advantages for biosensing applications. Firstly, the ability to create well-defined microdomains of TESUD with different terminal groups (-CHO or -COOH) allows for the selective immobilization of biomolecules. [] This is crucial for developing highly specific and sensitive biosensors. Secondly, TESUD SAMs are compatible with microfabrication techniques, enabling the development of miniaturized biosensors that can be integrated with existing technologies. []
Q4: Are there any limitations associated with using Triethoxysilylundecanal for surface modifications?
A: While TESUD offers various benefits, it's essential to consider potential limitations. The photooxidation process used to convert -CHO to -COOH groups requires controlled conditions to achieve the desired degree of modification without damaging the monolayer. [] Additionally, the stability of TESUD SAMs under different environmental conditions and prolonged use needs further investigation for specific applications.
Q5: What are the future directions for research on Triethoxysilylundecanal?
A5: Future research could focus on exploring the long-term stability and biocompatibility of TESUD-based SAMs for in vivo applications. Investigating the impact of different surface modification strategies on the performance of TESUD-based biosensors is another promising avenue. Furthermore, developing novel applications for TESUD beyond biosensing, such as in microfluidics or molecular electronics, holds significant potential.
Disclaimer and Information on In-Vitro Research Products
Please be aware that all articles and product information presented on BenchChem are intended solely for informational purposes. The products available for purchase on BenchChem are specifically designed for in-vitro studies, which are conducted outside of living organisms. In-vitro studies, derived from the Latin term "in glass," involve experiments performed in controlled laboratory settings using cells or tissues. It is important to note that these products are not categorized as medicines or drugs, and they have not received approval from the FDA for the prevention, treatment, or cure of any medical condition, ailment, or disease. We must emphasize that any form of bodily introduction of these products into humans or animals is strictly prohibited by law. It is essential to adhere to these guidelines to ensure compliance with legal and ethical standards in research and experimentation.