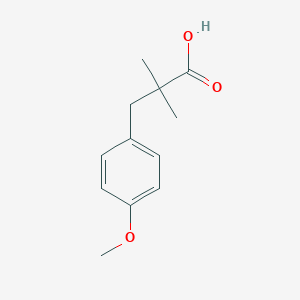
3-(4-Methoxyphenyl)-2,2-dimethylpropanoic acid
Overview
Description
Synthesis Analysis
The synthesis of 3-(4-Methoxyphenyl)-2,2-dimethylpropanoic acid and related compounds involves complex chemical reactions and methodologies. For example, Collins and Jacobs (1986) detailed the reaction of 1-methoxy-2-methyl-1-trimethylsilyloxyprop-1-ene with 1-acetoxy-1-(4′-methoxyphenyl)-2,2-dimethylpropane in the presence of zinc iodide, leading to various derivatives of the compound through hydride reduction and demethylation processes (Collins & Jacobs, 1986).
Molecular Structure Analysis
Studies such as those conducted by Düğdü et al. (2013) have focused on the structural characterization of 3-(4-Methoxyphenyl)-2,2-dimethylpropanoic acid derivatives. They utilized X-ray crystallography among other techniques to determine molecular geometry, demonstrating how structural elements influence the compound's properties (Düğdü et al., 2013).
Chemical Reactions and Properties
The chemical behavior of 3-(4-Methoxyphenyl)-2,2-dimethylpropanoic acid in reactions has been elucidated by several studies. For instance, Izquierdo and Carrasco (1984) described the reactivity of a similar compound, 3-(4-methoxyphenyl)-2-mercaptopropenoic acid, with nickel in alkaline media, highlighting its potential in spectrophotometric methods for nickel determination (Izquierdo & Carrasco, 1984).
Physical Properties Analysis
The physical properties of 3-(4-Methoxyphenyl)-2,2-dimethylpropanoic acid, such as crystalline structure and stability, are critical for understanding its potential applications. Katrusiak (1996) explored the crystal structure of a closely related compound, revealing insights into the conformational preferences and stability of the acidic protons in the crystalline state (Katrusiak, 1996).
Chemical Properties Analysis
The compound's interaction with other chemicals and its resulting chemical properties have been the focus of various research efforts. For example, the study by Ajibade and Andrew (2021) on derivatives synthesized via Schiff bases reduction route sheds light on the compound's potential for forming azo dyes and dithiocarbamate, providing a glimpse into its versatile chemical properties (Ajibade & Andrew, 2021).
Scientific Research Applications
Synthesis and Chemical Properties
3-(4-Methoxyphenyl)-2,2-dimethylpropanoic acid and its derivatives play a crucial role in the synthesis of complex organic compounds. For instance, the formation of cationic rhodium complexes with new tetraarylpentaborates demonstrates its utility in creating compounds with specific chemical properties, such as smooth hydrolysis to give distinct products (Nishihara, Nara, & Osakada, 2002). Additionally, the synthesis of the first 4-methoxy-substituted 1,3-benzazaphosphole highlights the compound's versatility in generating novel ligands with potential applications in various fields, including materials science (Aluri, Jones, Dix, & Heinicke, 2014).
Optoelectronic and Charge Transport Properties
In the realm of organic electronics, derivatives of 3-(4-Methoxyphenyl)-2,2-dimethylpropanoic acid have been explored for their optoelectronic and charge transport properties, making them promising candidates for use in organic light-emitting diodes (OLEDs). The study of dimethyl 2,3-bis((4-(bis(4-methoxyphenyl)amino)phenyl)ethynyl)fumarate and its acid-induced Pechmann dyes showcases their potential as efficient materials for OLEDs, based on DFT and TD-DFT calculations, as well as experimental observations (Wazzan & Irfan, 2019).
Anticancer and Antitumor Activities
The modification of 3-(4-Methoxyphenyl)-2,2-dimethylpropanoic acid to produce various derivatives has been linked to significant anticancer and antitumor activities. For instance, novel metal complexes derived from this compound have been synthesized and tested for their anti-tumor activities, showcasing potential as CDK8 kinase inhibitors in colon cancer therapy (Aboelmagd et al., 2021). Additionally, the synthesis of compounds based on structure modification of this acid has resulted in derivatives with potent HDAC inhibitory actions, offering new avenues for cancer treatment research (Rayes et al., 2020).
Antibacterial Activity
Research has also explored the antibacterial potential of derivatives of 3-(4-Methoxyphenyl)-2,2-dimethylpropanoic acid. For example, compounds synthesized from this acid have shown significant activity against various strains of microorganisms, highlighting their potential as new antibacterial agents with applications in combating infectious diseases (Osarumwense, 2022).
Mechanism of Action
Target of Action
It’s worth noting that compounds with similar structures, such as indole derivatives, have been found to bind with high affinity to multiple receptors , suggesting a potential for diverse biological interactions.
Mode of Action
Related compounds have been shown to interact with their targets in various ways, such as inhibiting or activating enzymatic activity, modulating receptor function, or altering cellular signaling pathways .
Biochemical Pathways
Related compounds have been shown to influence a variety of biochemical pathways, including those involved in inflammation, oxidative stress, and cell proliferation .
Pharmacokinetics
A related compound, 3-(4-hydroxy-3-methoxyphenyl)propionic acid, has been shown to undergo rapid metabolism and wide tissue distribution in sprague-dawley rats .
Result of Action
Related compounds have been shown to exert various biological activities, including antiviral, anti-inflammatory, anticancer, anti-hiv, antioxidant, antimicrobial, antitubercular, antidiabetic, antimalarial, and anticholinesterase activities .
Action Environment
It’s worth noting that the efficacy of similar compounds can be influenced by factors such as ph, temperature, and the presence of other substances .
Safety and Hazards
Future Directions
properties
IUPAC Name |
3-(4-methoxyphenyl)-2,2-dimethylpropanoic acid | |
---|---|---|
Source | PubChem | |
URL | https://pubchem.ncbi.nlm.nih.gov | |
Description | Data deposited in or computed by PubChem | |
InChI |
InChI=1S/C12H16O3/c1-12(2,11(13)14)8-9-4-6-10(15-3)7-5-9/h4-7H,8H2,1-3H3,(H,13,14) | |
Source | PubChem | |
URL | https://pubchem.ncbi.nlm.nih.gov | |
Description | Data deposited in or computed by PubChem | |
InChI Key |
NYDAEBWSGUNHIF-UHFFFAOYSA-N | |
Source | PubChem | |
URL | https://pubchem.ncbi.nlm.nih.gov | |
Description | Data deposited in or computed by PubChem | |
Canonical SMILES |
CC(C)(CC1=CC=C(C=C1)OC)C(=O)O | |
Source | PubChem | |
URL | https://pubchem.ncbi.nlm.nih.gov | |
Description | Data deposited in or computed by PubChem | |
Molecular Formula |
C12H16O3 | |
Source | PubChem | |
URL | https://pubchem.ncbi.nlm.nih.gov | |
Description | Data deposited in or computed by PubChem | |
Molecular Weight |
208.25 g/mol | |
Source | PubChem | |
URL | https://pubchem.ncbi.nlm.nih.gov | |
Description | Data deposited in or computed by PubChem | |
Product Name |
3-(4-Methoxyphenyl)-2,2-dimethylpropanoic acid |
Synthesis routes and methods I
Procedure details
Synthesis routes and methods II
Procedure details
Disclaimer and Information on In-Vitro Research Products
Please be aware that all articles and product information presented on BenchChem are intended solely for informational purposes. The products available for purchase on BenchChem are specifically designed for in-vitro studies, which are conducted outside of living organisms. In-vitro studies, derived from the Latin term "in glass," involve experiments performed in controlled laboratory settings using cells or tissues. It is important to note that these products are not categorized as medicines or drugs, and they have not received approval from the FDA for the prevention, treatment, or cure of any medical condition, ailment, or disease. We must emphasize that any form of bodily introduction of these products into humans or animals is strictly prohibited by law. It is essential to adhere to these guidelines to ensure compliance with legal and ethical standards in research and experimentation.