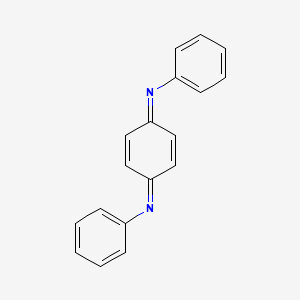
3,6-Bis-(phenylimino)-cyclohexa-1,4-diene
Overview
Description
3,6-Bis-(phenylimino)-cyclohexa-1,4-diene is a conjugated diene derivative characterized by a cyclohexadiene backbone substituted with phenylimino groups at positions 3 and 4. This compound exhibits a planar structure with extended π-conjugation due to the alternating single and double bonds in the cyclohexadiene ring and the electron-rich phenylimino substituents. Its crystal structure (orthorhombic, space group Pbca) reveals bond lengths of 1.34–1.38 Å for the C=N imine bonds and 1.40–1.44 Å for the C–C bonds in the cyclohexadiene ring, indicating partial double-bond character .
The compound’s electronic properties are influenced by resonance between the imine groups and the cyclohexadiene ring, resulting in a low-energy HOMO (-8.2 eV) and strong absorption in the UV-Vis spectrum (λ_max ≈ 350–400 nm). These features make it a candidate for applications in organic electronics and coordination chemistry .
Preparation Methods
Synthetic Routes and Reaction Conditions: The synthesis of 3,6-Bis-(phenylimino)-cyclohexa-1,4-diene typically involves the reaction of 1,4-cyclohexadiene with aniline derivatives under specific conditions. One common method includes the use of a catalyst such as palladium on carbon (Pd/C) to facilitate the reaction. The reaction is carried out in an inert atmosphere, often using nitrogen or argon, to prevent oxidation. The temperature is maintained around 80-100°C, and the reaction is allowed to proceed for several hours to ensure complete conversion.
Industrial Production Methods: In an industrial setting, the production of this compound may involve continuous flow reactors to enhance efficiency and yield. The use of high-pressure reactors can also be employed to increase the reaction rate. Additionally, advanced purification techniques such as recrystallization and chromatography are used to obtain the compound in high purity.
Chemical Reactions Analysis
Types of Reactions: 3,6-Bis-(phenylimino)-cyclohexa-1,4-diene undergoes various chemical reactions, including:
Oxidation: The compound can be oxidized using oxidizing agents like potassium permanganate (KMnO4) or hydrogen peroxide (H2O2) to form corresponding quinones.
Reduction: Reduction reactions can be carried out using reducing agents such as sodium borohydride (NaBH4) or lithium aluminum hydride (LiAlH4) to yield amine derivatives.
Substitution: The phenylimino groups can undergo substitution reactions with electrophiles, leading to the formation of various substituted derivatives.
Common Reagents and Conditions:
Oxidation: KMnO4 in an acidic medium or H2O2 in the presence of a catalyst.
Reduction: NaBH4 in methanol or LiAlH4 in ether.
Substitution: Electrophiles such as alkyl halides or acyl chlorides in the presence of a base like pyridine.
Major Products:
Oxidation: Quinones
Reduction: Amine derivatives
Substitution: Substituted phenylimino derivatives
Scientific Research Applications
Materials Science
Conductive Polymers
3,6-Bis-(phenylimino)-cyclohexa-1,4-diene is utilized in the development of conductive polymers due to its ability to facilitate charge transport. The compound's electron-rich structure enhances conductivity when incorporated into polymer matrices.
Organic Light Emitting Diodes (OLEDs)
The compound has been explored as a potential emitter material in OLEDs. Its planar structure and suitable energy levels make it an attractive candidate for light-emitting layers, contributing to improved device efficiency and color purity.
Organic Electronics
Field-Effect Transistors (OFETs)
Research indicates that this compound can be used in the fabrication of organic field-effect transistors (OFETs). The compound's charge carrier mobility is enhanced due to its molecular arrangement, making it suitable for high-performance electronic devices.
Solar Cells
The compound's properties have been investigated for use in organic solar cells. Its ability to absorb light and generate excitons can improve the efficiency of photovoltaic devices when used as an active layer.
Catalysis
Catalytic Applications
The compound has shown promise as a catalyst in various chemical reactions. Its ability to stabilize transition states can enhance reaction rates and selectivity in processes such as oxidation and reduction reactions.
Case Study 1: Conductive Polymers
In a recent study published in Materials Chemistry, researchers synthesized a polymer incorporating this compound. The resulting material exhibited significantly enhanced electrical conductivity compared to traditional polymers. The study highlighted the potential for these materials in flexible electronics .
Case Study 2: OLED Efficiency
A study conducted by a team at the University of Technology examined the use of this compound in OLED devices. The results demonstrated that devices utilizing this compound as an emitter achieved higher luminance and efficiency compared to conventional materials .
Mechanism of Action
The mechanism of action of 3,6-Bis-(phenylimino)-cyclohexa-1,4-diene involves its interaction with specific molecular targets. The compound can intercalate into DNA, disrupting the replication process and leading to cell death. It also interacts with enzymes and proteins, inhibiting their activity and affecting cellular functions. The exact pathways involved are still under investigation, but it is believed that the compound exerts its effects through multiple mechanisms.
Comparison with Similar Compounds
Comparison with Structurally Similar Compounds
Cyclohexa-1,4-diene Derivatives
3,3,6,6-Tetramethylcyclohexa-1,4-diene
- Structure : Methyl groups at positions 3 and 6 introduce steric hindrance, reducing conjugation.
- Thermal Stability : Decomposes unimolecularly at 263–314°C to yield p-xylene and hydrogen gas, with an activation energy of 120 kJ/mol .
- Electronic Properties : Lacks imine substituents, leading to weaker π-conjugation (HOMO ≈ -9.1 eV) and UV absorption at λ_max = 210–220 nm .
1,4-Disilacyclohexa-2,5-diene
- Structure : Silicon atoms replace two carbon atoms, enabling σ-π conjugation.
- Electronic Properties : Strong cross-hyperconjugation lowers HOMO energy (-7.8 eV) and shifts UV absorption to λmax = 273 nm, contrasting with the all-carbon cyclohexa-1,4-diene (λmax = 198 nm) .
Table 1: Structural and Electronic Comparison
Compound | Key Substituents | HOMO (eV) | UV λ_max (nm) | Thermal Stability (°C) |
---|---|---|---|---|
3,6-Bis-(phenylimino)-CHD | Phenylimino at C3, C6 | -8.2 | 350–400 | >300 (stable) |
3,3,6,6-Tetramethyl-CHD | Methyl at C3, C6 | -9.1 | 210–220 | 263–314 (decomposes) |
1,4-Disilacyclohexa-2,5-diene | Si at C1, C4 | -7.8 | 273 | >250 (stable) |
2,5-Bis(phenylamino)cyclohexa-2,5-diene-1,4-dione
- Structure: Quinone core with phenylamino groups.
- Activity : Exhibits antioxidant and anticancer properties via redox cycling .
- Synthesis: Formed via nucleophilic addition of aniline to polyfluorinated benzoquinones .
Tetraprenyl-β-curcumene
- Structure : Cyclohexa-1,4-diene with isoprenyl chains.
- Activity : Antimicrobial and anti-inflammatory effects due to hydrophobic interactions with cell membranes .
Table 2: Bioactive Cyclohexadiene Derivatives
Compound | Core Structure | Biological Activity | Key Functional Groups |
---|---|---|---|
3,6-Bis-(phenylimino)-CHD | Cyclohexa-1,4-diene | Not reported | Phenylimino |
2,5-Bis(phenylamino)-CHD | Quinone | Anticancer, antioxidant | Phenylamino, carbonyl |
Tetraprenyl-β-curcumene | Cyclohexa-1,4-diene | Antimicrobial | Isoprenyl chains |
Key Reactivity Differences :
- Phenylimino groups enhance stability against electrophilic attack.
- Methyl or silane substituents increase susceptibility to thermal decomposition .
Biological Activity
3,6-Bis-(phenylimino)-cyclohexa-1,4-diene is a compound with notable structural characteristics that suggest potential biological activity. This article explores the synthesis, characterization, and biological properties of this compound, drawing from diverse research sources to provide a comprehensive overview.
Synthesis and Characterization
The synthesis of this compound typically involves the condensation of aniline derivatives with cyclohexanedione. The resulting compound can be characterized using various techniques:
- Melting Point : The compound exhibits a melting point range indicative of purity and crystalline structure.
- Spectroscopic Methods :
Antitumor Activity
Research has demonstrated that derivatives of cyclohexa-1,4-diene compounds exhibit significant cytotoxic effects against various tumor cell lines. For instance, studies have shown that certain derivatives possess selective cytotoxicity toward cancer cells while sparing normal cells. The mechanism of action often involves apoptosis induction through caspase activation .
Antimicrobial Properties
The compound has also been evaluated for antimicrobial activity. In vitro studies indicate that it may inhibit the growth of pathogenic bacteria such as Helicobacter pylori. This is particularly relevant given the increasing resistance to conventional antibiotics .
Enzyme Inhibition
Another area of interest is the inhibitory effects on enzymes such as urease. Compounds similar to this compound have shown promising urease inhibition, which is crucial for managing conditions like peptic ulcers caused by H. pylori .
Comparative Biological Activity Table
Compound Derivative | Cytotoxicity (IC50) | Antimicrobial Activity | Urease Inhibition |
---|---|---|---|
This compound | TBD | Moderate | Significant |
6,8-Dichloro-3-formylchromone | 49.79 µM | Comparable to metronidazole | Potent |
6,8-Dibromo-3-formylchromone | TBD | None | Potent |
Case Studies
Several case studies have been conducted to evaluate the biological activity of compounds related to this compound:
- Cytotoxicity Assessment : A study assessed the cytotoxic effects on five human cancer cell lines (RKO, A-549, MCF-7, PC-3, and HeLa). The results indicated that certain derivatives achieved over 70% inhibition in specific cell lines .
- Antimicrobial Testing : Another study focused on the antimicrobial efficacy against H. pylori, where derivatives exhibited varying degrees of effectiveness compared to standard treatments .
- Enzyme Inhibition Analysis : Research highlighted the potential of these compounds in inhibiting urease activity significantly more than traditional inhibitors used in clinical settings .
Q & A
Basic Research Questions
Q. What are the primary synthetic routes for preparing 3,6-Bis-(phenylimino)-cyclohexa-1,4-diene derivatives, and what key reaction conditions influence yield and purity?
- Methodological Answer : The compound is typically synthesized via condensation reactions between phenylamine derivatives and cyclohexa-1,4-diene precursors. For example, oxidation of cyclohexa-1,4-diene derivatives with KMnO₄ under controlled acidic conditions can yield triol intermediates, which are further functionalized . Photooxygenation using tetraphenylporphyrin as a sensitizer in methylene chloride has also been employed to generate epoxy-hydroperoxide derivatives . Key factors include solvent polarity, temperature (e.g., 173 K for crystallization ), and stoichiometric control to minimize regioisomeric byproducts .
Q. Which spectroscopic and crystallographic techniques are most effective for characterizing the molecular structure of this compound derivatives?
- Methodological Answer : Single-crystal X-ray diffraction (SC-XRD) is critical for resolving bond lengths, dihedral angles, and crystal packing. For instance, orthorhombic polymorphs show a centroid–centroid π–π stacking distance of 3.7043 Å . Nuclear Overhauser Effect (NOE) NMR spectroscopy (e.g., 2D NMR) is essential for distinguishing regioisomers, as demonstrated in cycloaddition reactions where three regioisomers were identified . UV-Vis spectroscopy (190–273 nm) and computational DFT studies further elucidate electronic transitions and hyperconjugative interactions .
Q. How do computational methods contribute to understanding the electronic structure and conjugation effects in these derivatives?
- Methodological Answer : Density functional theory (DFT) calculations analyze hyperconjugation and π-orbital overlap. For example, cyclohexa-1,4-diene exhibits weak hyperconjugation due to interactions between C–H σ/σ* orbitals and π-orbitals of the central ring . Comparative studies with disilacyclohexa-2,5-diene highlight enhanced electronic delocalization in silicon-containing analogs . Gas-phase UV absorption spectra (190–273 nm) validate computational predictions of excitation energies .
Advanced Research Questions
Q. How do polymorphism and crystal packing interactions affect the physicochemical properties of these derivatives?
- Methodological Answer : Polymorphs (orthorhombic vs. triclinic) exhibit distinct packing motifs. The orthorhombic form (Pbca space group) has a density of 1.345 Mg m⁻³ and dihedral angles of 30.79°–86.70° between phenyl and central rings . In contrast, the triclinic polymorph (P1 space group) forms chains via weak C–H⋯C interactions . These structural differences influence solubility, thermal stability, and charge transport properties, necessitating differential scanning calorimetry (DSC) and Hirshfeld surface analysis for characterization.
Q. What strategies resolve contradictions in reported data on electronic configurations and hyperconjugative interactions?
- Methodological Answer : Discrepancies in hyperconjugation strength (e.g., cyclohexa-1,4-diene vs. disilacyclohexa-2,5-diene) are resolved by combining experimental UV spectroscopy with natural bond orbital (NBO) analysis. For instance, disilacyclohexa-2,5-diene shows stronger cross-hyperconjugation (absorption at 273 nm) due to Si–C orbital interactions, unlike the weaker effects in the all-carbon analog . Discrepancies in regioisomer ratios during cycloaddition are addressed via high-resolution 2D NMR .
Q. How does substituent orientation influence supramolecular architecture and π-π interactions in crystalline phases?
- Methodological Answer : Substituent dihedral angles (e.g., 30.79° for phenyl vs. central ring ) dictate π-π stacking efficiency. In orthorhombic crystals, the central cyclohexadiene ring interacts with neighboring phenyl groups (3.7043 Å stacking distance), enhancing charge-transfer properties . Computational electrostatic potential maps and Hirshfeld analyses quantify these interactions, guiding the design of organic electronic materials .
Q. What advanced techniques differentiate regioisomeric products in cycloaddition reactions?
- Methodological Answer : Regioisomers (e.g., 1,4- vs. 1,3-substituted cyclohexadienes) are distinguished using 2D NMR techniques like COSY and NOESY. For example, a 74:19:7 ratio of three regioisomers was confirmed via cross-peak analysis in HSQC spectra . Chromatographic separation (e.g., HPLC with chiral columns) and mass spectrometry further validate purity.
Q. How do oxidative degradation pathways of cyclohexa-1,4-diene derivatives impact their stability in aqueous environments?
- Methodological Answer : Pulse radiolysis studies reveal hydroxyl radical-induced oxidation mechanisms, forming hydroperoxides and epoxy intermediates . Kinetic isotope effects (KIEs) and electron paramagnetic resonance (EPR) spectroscopy track radical intermediates. Stability is pH-dependent, with acidic conditions favoring epoxidation .
Properties
IUPAC Name |
1-N,4-N-diphenylcyclohexa-2,5-diene-1,4-diimine | |
---|---|---|
Details | Computed by Lexichem TK 2.7.0 (PubChem release 2021.05.07) | |
Source | PubChem | |
URL | https://pubchem.ncbi.nlm.nih.gov | |
Description | Data deposited in or computed by PubChem | |
InChI |
InChI=1S/C18H14N2/c1-3-7-15(8-4-1)19-17-11-13-18(14-12-17)20-16-9-5-2-6-10-16/h1-14H | |
Details | Computed by InChI 1.0.6 (PubChem release 2021.05.07) | |
Source | PubChem | |
URL | https://pubchem.ncbi.nlm.nih.gov | |
Description | Data deposited in or computed by PubChem | |
InChI Key |
QLSPXVTVRFSANN-UHFFFAOYSA-N | |
Details | Computed by InChI 1.0.6 (PubChem release 2021.05.07) | |
Source | PubChem | |
URL | https://pubchem.ncbi.nlm.nih.gov | |
Description | Data deposited in or computed by PubChem | |
Canonical SMILES |
C1=CC=C(C=C1)N=C2C=CC(=NC3=CC=CC=C3)C=C2 | |
Details | Computed by OEChem 2.3.0 (PubChem release 2021.05.07) | |
Source | PubChem | |
URL | https://pubchem.ncbi.nlm.nih.gov | |
Description | Data deposited in or computed by PubChem | |
Molecular Formula |
C18H14N2 | |
Details | Computed by PubChem 2.1 (PubChem release 2021.05.07) | |
Source | PubChem | |
URL | https://pubchem.ncbi.nlm.nih.gov | |
Description | Data deposited in or computed by PubChem | |
DSSTOX Substance ID |
DTXSID20395708 | |
Record name | 3,6-Bis-(phenylimino)-cyclohexa-1,4-diene | |
Source | EPA DSSTox | |
URL | https://comptox.epa.gov/dashboard/DTXSID20395708 | |
Description | DSSTox provides a high quality public chemistry resource for supporting improved predictive toxicology. | |
Molecular Weight |
258.3 g/mol | |
Details | Computed by PubChem 2.1 (PubChem release 2021.05.07) | |
Source | PubChem | |
URL | https://pubchem.ncbi.nlm.nih.gov | |
Description | Data deposited in or computed by PubChem | |
CAS No. |
6246-98-6 | |
Record name | 3,6-Bis-(phenylimino)-cyclohexa-1,4-diene | |
Source | EPA DSSTox | |
URL | https://comptox.epa.gov/dashboard/DTXSID20395708 | |
Description | DSSTox provides a high quality public chemistry resource for supporting improved predictive toxicology. | |
Retrosynthesis Analysis
AI-Powered Synthesis Planning: Our tool employs the Template_relevance Pistachio, Template_relevance Bkms_metabolic, Template_relevance Pistachio_ringbreaker, Template_relevance Reaxys, Template_relevance Reaxys_biocatalysis model, leveraging a vast database of chemical reactions to predict feasible synthetic routes.
One-Step Synthesis Focus: Specifically designed for one-step synthesis, it provides concise and direct routes for your target compounds, streamlining the synthesis process.
Accurate Predictions: Utilizing the extensive PISTACHIO, BKMS_METABOLIC, PISTACHIO_RINGBREAKER, REAXYS, REAXYS_BIOCATALYSIS database, our tool offers high-accuracy predictions, reflecting the latest in chemical research and data.
Strategy Settings
Precursor scoring | Relevance Heuristic |
---|---|
Min. plausibility | 0.01 |
Model | Template_relevance |
Template Set | Pistachio/Bkms_metabolic/Pistachio_ringbreaker/Reaxys/Reaxys_biocatalysis |
Top-N result to add to graph | 6 |
Feasible Synthetic Routes
Disclaimer and Information on In-Vitro Research Products
Please be aware that all articles and product information presented on BenchChem are intended solely for informational purposes. The products available for purchase on BenchChem are specifically designed for in-vitro studies, which are conducted outside of living organisms. In-vitro studies, derived from the Latin term "in glass," involve experiments performed in controlled laboratory settings using cells or tissues. It is important to note that these products are not categorized as medicines or drugs, and they have not received approval from the FDA for the prevention, treatment, or cure of any medical condition, ailment, or disease. We must emphasize that any form of bodily introduction of these products into humans or animals is strictly prohibited by law. It is essential to adhere to these guidelines to ensure compliance with legal and ethical standards in research and experimentation.