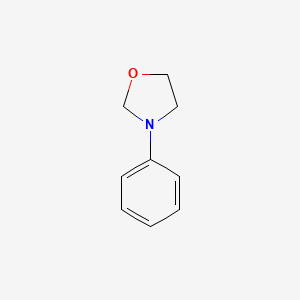
3-Phenyloxazolidine
Overview
Description
3-Phenyloxazolidine is a heterocyclic organic compound characterized by a five-membered oxazolidine ring (containing one oxygen and one nitrogen atom) substituted with a phenyl group at the 3-position. Its synthesis has been reported via pyrolysis of specific urea derivatives, yielding 2-(phenylimino)-3-phenyloxazolidine and related analogs . This compound has garnered attention in computational chemistry studies due to challenges in predicting its C–H bond dissociation enthalpy (BDE), a critical parameter for understanding reactivity and stability in organic and medicinal chemistry .
Preparation Methods
3-Phenyloxazolidine can be synthesized through several methods. One common synthetic route involves the reaction of 1,2-amino alcohols with aldehydes or ketones under acidic or basic conditions. For example, the reaction of phenylglycinol with formaldehyde in the presence of an acid catalyst can yield this compound . Industrial production methods may involve multicomponent reactions, such as the Mannich reaction, which allows for the efficient synthesis of functionalized oxazolidines .
Chemical Reactions Analysis
3-Phenyloxazolidine undergoes various chemical reactions, including:
Oxidation: It can be oxidized to form oxazolidinones, which are important intermediates in the synthesis of antibiotics.
Reduction: Reduction reactions can convert oxazolidines to amino alcohols.
Substitution: The phenyl group can undergo electrophilic aromatic substitution reactions, allowing for further functionalization of the compound. Common reagents used in these reactions include oxidizing agents like hydrogen peroxide and reducing agents such as sodium borohydride.
Scientific Research Applications
Medicinal Chemistry Applications
3-Phenyloxazolidine and its derivatives have been studied extensively for their potential therapeutic effects, particularly in the development of antibiotics and anti-inflammatory agents.
Antibiotic Development
One of the notable applications of this compound is in the synthesis of oxazolidinone antibiotics. These compounds are effective against Gram-positive bacteria, including resistant strains like MRSA (Methicillin-resistant Staphylococcus aureus). A study demonstrated that derivatives of this compound showed significant antibacterial activity, making them promising candidates for new antibiotic therapies .
Anti-Inflammatory Properties
Research has indicated that this compound exhibits anti-inflammatory properties, which can be beneficial in treating conditions such as psoriasis. Network pharmacology studies have identified multiple targets and pathways through which this compound may exert its effects, suggesting a multi-target approach to treatment .
Agricultural Applications
In agriculture, this compound derivatives have shown efficacy as fungicides and bactericides.
Seed Treatment for Disease Control
A patent describes the use of 5-(chloromethyl)-3-phenyloxazolidinone as a seed treatment to control diseases caused by pathogens such as Pythium spp. and Agrobacterium tumefaciens. In greenhouse trials, treated cotton seeds demonstrated an 88% emergence rate compared to untreated seeds, which failed to germinate in infected soil . This indicates the potential of this compound-based compounds in enhancing crop resilience against diseases.
Bacterial Disease Management
Further studies have shown that formulations containing this compound can effectively manage bacterial diseases in crops like tomatoes. When applied as a foliar spray, these compounds significantly reduced disease severity compared to untreated controls .
Synthesis and Catalysis
This compound is also utilized in synthetic chemistry as a chiral auxiliary or catalyst in asymmetric synthesis.
Asymmetric Synthesis
Research has highlighted the role of this compound in the asymmetric aminolysis of epoxides. The development of chiral macrocyclic metal complexes using this compound has led to high enantiomeric excess in product yields, showcasing its utility in producing enantiopure compounds .
Chiral Auxiliary Applications
The use of oxazolidines as chiral auxiliaries has been well-documented, with this compound derivatives facilitating enantioselective reactions in total synthesis pathways for various biologically active compounds .
Data Summary
The following table summarizes key findings related to the applications of this compound:
Mechanism of Action
The mechanism of action of 3-Phenyloxazolidine and its derivatives often involves the inhibition of protein synthesis. For example, oxazolidinone antibiotics inhibit bacterial protein synthesis by binding to the 50S ribosomal subunit, preventing the formation of a functional 70S initiation complex . This unique mechanism makes them effective against a wide range of Gram-positive pathogens.
Comparison with Similar Compounds
Comparison with Similar Compounds
C–H Bond Dissociation Enthalpy (BDE) Prediction Accuracy
A machine learning-enhanced semi-empirical quantum mechanics study evaluated 50 compounds for C–H BDE prediction accuracy. 3-Phenyloxazolidine (Compound 8) exhibited significant errors compared to other derivatives:
Compound | rPM6/ML Error (kcal/mol) | GFN2-xTB/ML Error (kcal/mol) |
---|---|---|
This compound | 5.7 | 6.4 |
Compound 5 (Unspecified) | 7.0 | 5.8 |
Other Compounds (32/50) | ≤1.0 | ≤1.0 |
This compound’s high errors (5.7–6.4 kcal/mol) contrast with 32 compounds achieving sub-1.0 kcal/mol accuracy, highlighting its unique electronic or steric effects. The phenyl substituent likely induces resonance or conformational strain, complicating BDE predictions .
Structural Analogs and Substituent Effects
Synthetic analogs of this compound, such as 2-(phenylimino)-3-phenyloxazolidine, demonstrate how substituent variations impact properties. For example:
- Pyrolysis of N-substituted ureas to form these analogs suggests distinct synthetic pathways compared to simpler oxazolidines .
- Phenyl Group Position : Derivatives with phenyl groups at alternative positions (e.g., 2- or 4-positions) remain unexplored in the provided data but could offer insights into regiochemical influences on BDE or solubility.
Comparison with Other Oxazolidine Derivatives
- Synthetic Accessibility: this compound requires specialized pyrolysis conditions , whereas simpler oxazolidines may form via cyclization of amino alcohols under milder conditions.
Research Findings and Implications
- Computational Challenges : The poor BDE prediction accuracy for this compound underscores the need for advanced quantum mechanical methods or hybrid models to capture its electronic complexity .
- Design Considerations : Substituent choice (e.g., aryl vs. alkyl groups) significantly impacts oxazolidine properties, guiding the design of analogs for pharmaceutical or materials science applications.
- Synthetic Optimization : Pyrolysis-based routes for phenyl-substituted oxazolidines may require optimization to improve yields or purity compared to conventional methods .
Biological Activity
3-Phenyloxazolidine is a compound that has garnered attention in the field of medicinal chemistry due to its diverse biological activities. This article explores the biological activity of this compound and its derivatives, focusing on their mechanisms of action, therapeutic potentials, and structure-activity relationships (SAR).
Chemical Structure
This compound belongs to the oxazolidine family, characterized by a five-membered heterocyclic ring containing nitrogen and oxygen. The general structure can be represented as follows:
This structure allows for various substitutions that can significantly influence its biological activity.
Antimicrobial Properties
Research indicates that derivatives of this compound exhibit significant antimicrobial activities. A notable study highlighted the fungicidal action of 3-phenyl-oxazolidine-2,4-dione derivatives, which demonstrated effectiveness against a variety of phytopathogenic fungi. These compounds can be halogenated to enhance their microbicidal properties, showing a broader spectrum of activity compared to previously known compounds .
Antiviral Activity
This compound derivatives have also been explored as potential inhibitors of HIV-1 protease. A series of new inhibitors were designed with varying phenyloxazolidinone P2 ligands, demonstrating low nanomolar antiviral potency against multiple HIV-1 clades. The binding affinity was found to be significantly affected by the position of substitutions on the phenyl ring, with 3-substituted analogues generally exhibiting superior binding properties compared to 2- and 4-substituted ones .
Antioxidant and Antiplatelet Activity
Recent studies have identified 3-phenyl-1H-isochromen-1-one analogues as potent antioxidants and antiplatelet agents. These compounds displayed antioxidant activities significantly greater than ascorbic acid and exhibited strong inhibition of arachidonic acid-induced platelet aggregation, surpassing traditional agents like aspirin .
Cardiovascular Effects
In vivo studies on certain phenyloxazolidines have shown promising antihypertensive effects. For instance, experiments indicated that specific doses led to significant increases in blood pressure and heart rate in animal models, suggesting potential applications in cardiovascular therapeutics .
Structure-Activity Relationships (SAR)
Understanding the SAR is crucial for optimizing the biological activity of this compound derivatives. Key findings include:
- Substitution Patterns : The position of substituents on the phenyl ring greatly influences the compound's binding affinity and biological efficacy.
- Optical Isomers : Different optical isomers of these compounds exhibit varying degrees of antimicrobial activity, highlighting the importance of stereochemistry in drug design .
Table: Summary of Biological Activities
Case Study 1: Antimicrobial Efficacy
A series of experiments conducted on various this compound derivatives revealed that halogenated compounds exhibited enhanced antifungal properties. The study demonstrated a marked reduction in fungal growth at specific concentrations, indicating their potential as effective agricultural fungicides.
Case Study 2: HIV Protease Inhibition
A focused investigation into the binding affinities of different derivatives led to the discovery of several compounds with pM binding affinities. These inhibitors were tested against patient-derived viruses, showing promising results in terms of both potency and resistance profiles.
Q & A
Basic Research Questions
Q. What are the established synthetic routes for 3-phenyloxazolidine, and how do reaction conditions influence yield and purity?
- Methodological Answer : Synthesis often involves cyclization of β-amino alcohols or decomposition of urea derivatives (e.g., pyrolysis of N-carbamyl-substituted ureas). Optimization requires adjusting catalysts (e.g., acidic/basic conditions), temperature, and solvent polarity. For example, pyrolysis of N-(carbamyl)ethyl-N,N'-diphenylurea under controlled heating (150–200°C) yields 2-(phenylimino)-3-phenyloxazolidine, but side products may form if reaction time exceeds optimal thresholds . Characterization via <sup>1</sup>H/<sup>13</sup>C NMR and GC-MS is critical to confirm purity.
Q. How can researchers validate the structural stability of this compound under varying experimental conditions?
- Methodological Answer : Stability studies should employ thermogravimetric analysis (TGA) and differential scanning calorimetry (DSC) to assess thermal degradation thresholds. For hydrolytic stability, incubate the compound in buffers of varying pH (e.g., pH 3–10) and monitor decomposition via HPLC. Comparative studies with analogs (e.g., 2-sulfonyloxaziridines) reveal electron-withdrawing substituents enhance stability by reducing nucleophilic attack at the oxazolidine ring .
Q. What spectroscopic techniques are most reliable for characterizing this compound derivatives?
- Methodological Answer : Use a combination of <sup>15</sup>N NMR to probe nitrogen environments and IR spectroscopy to identify carbonyl or imine stretches. X-ray crystallography resolves stereochemical ambiguities, particularly for chiral oxazolidines. For example, (±)-trans-2-(phenylsulfonyl)-3-phenyloxaziridine’s crystal structure confirmed its stereochemistry via bond angles and torsion parameters .
Advanced Research Questions
Q. How do computational methods (e.g., DFT or machine learning) improve predictions of this compound’s reactivity and thermodynamic properties?
- Methodological Answer : Density functional theory (DFT) calculates bond dissociation energies (BDEs) and transition states for ring-opening reactions. Machine learning (ML) models, trained on datasets like C–H BDEs of heterocycles, reduce errors in semi-empirical methods (e.g., GFN2-xTB overestimates BDEs by 7–8 kcal/mol for 3-phenyloxazridines). Integrating ML with rPM6 improves MAEs to ≤5 kcal/mol, enabling accurate reactivity predictions .
Q. What experimental strategies resolve contradictions in reported catalytic activities of this compound derivatives?
- Methodological Answer : Apply systematic review frameworks (e.g., PRISMA) to identify bias in literature. For instance, discrepancies in enantioselective catalysis may arise from unaccounted solvent effects or impurities. Replicate studies under standardized conditions (e.g., inert atmosphere, anhydrous solvents) and use control experiments (e.g., kinetic isotopic effects) to isolate variables. Meta-analyses of kinetic data can clarify trends .
Q. How can researchers design comparative studies to evaluate this compound’s bioactivity against structurally related heterocycles?
- Methodological Answer : Use the PICO framework:
- Population : Target enzyme/receptor (e.g., cytochrome P450).
- Intervention : this compound and analogs (e.g., oxaziridines, isoxazolines).
- Comparison : IC50, binding affinity, or metabolic half-life.
- Outcome : Structure-activity relationships (SARs).
High-throughput screening with dose-response assays and molecular docking validates hypotheses about substituent effects on bioactivity .
Q. Data Analysis and Interpretation
Q. What statistical approaches are optimal for analyzing nonlinear kinetics in this compound-mediated reactions?
- Methodological Answer : Use non-linear regression (e.g., Michaelis-Menten or Hill equations) to model enzyme-like catalysis. Bootstrap resampling quantifies confidence intervals for kinetic parameters (kcat, KM). For autocatalytic pathways, time-series analysis (e.g., Avrami kinetics) identifies nucleation-growth mechanisms .
Q. How should researchers address reproducibility challenges in synthesizing enantiopure this compound derivatives?
- Methodological Answer : Implement chiral chromatography (e.g., HPLC with amylose-based columns) and circular dichroism (CD) to verify enantiomeric excess (ee). Document synthetic protocols with granular detail (e.g., solvent batch, stirring rate) using FAIR data principles. Collaborative inter-laboratory studies identify critical control points (e.g., moisture sensitivity) .
Properties
IUPAC Name |
3-phenyl-1,3-oxazolidine | |
---|---|---|
Details | Computed by Lexichem TK 2.7.0 (PubChem release 2021.05.07) | |
Source | PubChem | |
URL | https://pubchem.ncbi.nlm.nih.gov | |
Description | Data deposited in or computed by PubChem | |
InChI |
InChI=1S/C9H11NO/c1-2-4-9(5-3-1)10-6-7-11-8-10/h1-5H,6-8H2 | |
Details | Computed by InChI 1.0.6 (PubChem release 2021.05.07) | |
Source | PubChem | |
URL | https://pubchem.ncbi.nlm.nih.gov | |
Description | Data deposited in or computed by PubChem | |
InChI Key |
ITSRLVMSQHOSEC-UHFFFAOYSA-N | |
Details | Computed by InChI 1.0.6 (PubChem release 2021.05.07) | |
Source | PubChem | |
URL | https://pubchem.ncbi.nlm.nih.gov | |
Description | Data deposited in or computed by PubChem | |
Canonical SMILES |
C1COCN1C2=CC=CC=C2 | |
Details | Computed by OEChem 2.3.0 (PubChem release 2021.05.07) | |
Source | PubChem | |
URL | https://pubchem.ncbi.nlm.nih.gov | |
Description | Data deposited in or computed by PubChem | |
Molecular Formula |
C9H11NO | |
Details | Computed by PubChem 2.1 (PubChem release 2021.05.07) | |
Source | PubChem | |
URL | https://pubchem.ncbi.nlm.nih.gov | |
Description | Data deposited in or computed by PubChem | |
DSSTOX Substance ID |
DTXSID10334141 | |
Record name | 3-Phenyloxazolidine | |
Source | EPA DSSTox | |
URL | https://comptox.epa.gov/dashboard/DTXSID10334141 | |
Description | DSSTox provides a high quality public chemistry resource for supporting improved predictive toxicology. | |
Molecular Weight |
149.19 g/mol | |
Details | Computed by PubChem 2.1 (PubChem release 2021.05.07) | |
Source | PubChem | |
URL | https://pubchem.ncbi.nlm.nih.gov | |
Description | Data deposited in or computed by PubChem | |
CAS No. |
20503-92-8 | |
Record name | 3-Phenyloxazolidine | |
Source | EPA DSSTox | |
URL | https://comptox.epa.gov/dashboard/DTXSID10334141 | |
Description | DSSTox provides a high quality public chemistry resource for supporting improved predictive toxicology. | |
Synthesis routes and methods
Procedure details
Retrosynthesis Analysis
AI-Powered Synthesis Planning: Our tool employs the Template_relevance Pistachio, Template_relevance Bkms_metabolic, Template_relevance Pistachio_ringbreaker, Template_relevance Reaxys, Template_relevance Reaxys_biocatalysis model, leveraging a vast database of chemical reactions to predict feasible synthetic routes.
One-Step Synthesis Focus: Specifically designed for one-step synthesis, it provides concise and direct routes for your target compounds, streamlining the synthesis process.
Accurate Predictions: Utilizing the extensive PISTACHIO, BKMS_METABOLIC, PISTACHIO_RINGBREAKER, REAXYS, REAXYS_BIOCATALYSIS database, our tool offers high-accuracy predictions, reflecting the latest in chemical research and data.
Strategy Settings
Precursor scoring | Relevance Heuristic |
---|---|
Min. plausibility | 0.01 |
Model | Template_relevance |
Template Set | Pistachio/Bkms_metabolic/Pistachio_ringbreaker/Reaxys/Reaxys_biocatalysis |
Top-N result to add to graph | 6 |
Feasible Synthetic Routes
Disclaimer and Information on In-Vitro Research Products
Please be aware that all articles and product information presented on BenchChem are intended solely for informational purposes. The products available for purchase on BenchChem are specifically designed for in-vitro studies, which are conducted outside of living organisms. In-vitro studies, derived from the Latin term "in glass," involve experiments performed in controlled laboratory settings using cells or tissues. It is important to note that these products are not categorized as medicines or drugs, and they have not received approval from the FDA for the prevention, treatment, or cure of any medical condition, ailment, or disease. We must emphasize that any form of bodily introduction of these products into humans or animals is strictly prohibited by law. It is essential to adhere to these guidelines to ensure compliance with legal and ethical standards in research and experimentation.