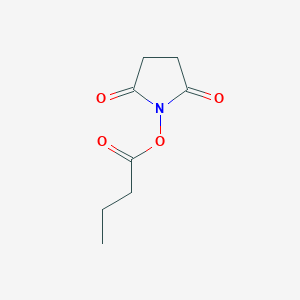
butyric acid NHS ester
Overview
Description
Butyric acid N-hydroxysuccinimide ester is a chemical compound that combines butyric acid with N-hydroxysuccinimide. This ester is commonly used in bioconjugation and crosslinking applications due to its ability to react with primary amines to form stable amide bonds. This property makes it valuable in various fields, including biochemistry, molecular biology, and medical research.
Mechanism of Action
Target of Action
The primary targets of Butyric Acid NHS Ester are primary amines . These exist at the N-terminus of each polypeptide chain and in the side-chain of lysine (Lys, K) amino acid residues . These primary amines are positively charged at physiologic pH and occur predominantly on the outside surfaces of native protein tertiary structures, making them readily accessible to conjugation reagents .
Mode of Action
This compound interacts with its targets by forming a chemically stable amide bond . The reaction is strongly pH-dependent, mainly due to the varying degrees of the protonation of the amine at each pH .
Biochemical Pathways
The biochemical pathway involved in the action of this compound is the amine-reactive crosslinker pathway . This pathway involves the use of chemical groups that react with primary amines for crosslinking or labeling peptides and proteins . The reaction leads to the creation of a stable amide bond between the labeling reagent and the target molecule .
Pharmacokinetics
It’s known that the reaction of nhs esters with amines is ph-dependent , which could potentially impact the bioavailability of the compound.
Result of Action
The result of the action of this compound is the formation of a stable amide bond between the labeling reagent and the target molecule . This allows for the labeling and crosslinking of peptides and proteins, such as antibodies .
Action Environment
The action of this compound is influenced by environmental factors such as pH and the presence of water or moisture . The reaction is strongly pH-dependent, with deprotonated primary amines being the most nucleophilic . Furthermore, NHS esters are activated through spontaneous hydrolysis in the presence of water and/or moisture .
Biochemical Analysis
Biochemical Properties
Butyric acid N-hydroxysuccinimide ester is known for its ability to react with primary amines, which are common functional groups present in proteins, peptides, and other macromolecules . This interaction occurs at the N-terminus of each polypeptide chain and in the side-chain of lysine amino acid residues .
Cellular Effects
The cellular effects of butyric acid N-hydroxysuccinimide ester are primarily due to its interactions with primary amines. These amines are positively charged at physiological pH and occur predominantly on the outside surfaces of native protein tertiary structures . This makes them readily accessible to conjugation reagents like butyric acid N-hydroxysuccinimide ester introduced into the aqueous medium .
Molecular Mechanism
The molecular mechanism of action of butyric acid N-hydroxysuccinimide ester involves the formation of chemical bonds with primary amines. These include isothiocyanates, isocyanates, acyl azides, NHS esters, sulfonyl chlorides, aldehydes, glyoxals, epoxides, oxiranes, carbonates, aryl halides, imidoesters, carbodiimides, anhydrides, and fluorophenyl esters .
Temporal Effects in Laboratory Settings
The extent of NHS-ester hydrolysis in aqueous solutions free of primary amines can be measured at 260 to 280 nm, because the NHS byproduct absorbs in that range . NHS-ester crosslinking reactions are most commonly performed in phosphate, carbonate-bicarbonate, HEPES or borate buffers at pH 7.2 to 8.5 for 0.5 to 4 h at room temperature or 4°C .
Metabolic Pathways
Butyric acid N-hydroxysuccinimide ester is involved in the metabolic pathways that involve primary amines. These amines are especially nucleophilic, making them easy targets for conjugation with several reactive groups .
Preparation Methods
Synthetic Routes and Reaction Conditions: The synthesis of butyric acid N-hydroxysuccinimide ester typically involves the reaction of butyric acid with N-hydroxysuccinimide in the presence of a coupling reagent such as dicyclohexylcarbodiimide (DCC) or 1-ethyl-3-(3-dimethylaminopropyl)carbodiimide (EDC). The reaction is carried out in an anhydrous solvent, often dichloromethane or dimethylformamide (DMF), under mild conditions. The resulting ester can be purified by recrystallization or chromatography.
Industrial Production Methods: In industrial settings, the production of butyric acid N-hydroxysuccinimide ester follows similar principles but on a larger scale. The process involves the use of automated reactors and precise control of reaction parameters to ensure high yield and purity. The ester is then isolated and purified using industrial-scale chromatography or crystallization techniques.
Chemical Reactions Analysis
Types of Reactions: Butyric acid N-hydroxysuccinimide ester primarily undergoes nucleophilic substitution reactions with primary amines. This reaction forms a stable amide bond and releases N-hydroxysuccinimide as a byproduct. The ester can also participate in hydrolysis reactions, especially in the presence of water, leading to the formation of butyric acid and N-hydroxysuccinimide.
Common Reagents and Conditions:
Nucleophilic Substitution: Primary amines (e.g., lysine residues in proteins) in a slightly alkaline buffer (pH 7.2 to 9) are common reagents. The reaction is typically carried out at room temperature.
Hydrolysis: Water or aqueous buffers can hydrolyze the ester, especially under acidic or basic conditions.
Major Products:
Amide Formation: The primary product of the reaction with amines is a stable amide bond.
Hydrolysis Products: Butyric acid and N-hydroxysuccinimide.
Scientific Research Applications
Butyric acid N-hydroxysuccinimide ester is widely used in scientific research due to its versatility and reactivity. Some key applications include:
Protein Labeling and Crosslinking: It is used to label proteins with various tags or to crosslink proteins for structural studies.
Bioconjugation: The ester is employed in the conjugation of biomolecules, such as attaching fluorescent dyes to antibodies for imaging applications.
Drug Delivery: It is used in the development of drug delivery systems where the ester facilitates the attachment of therapeutic agents to targeting molecules.
Surface Modification: The ester is used to modify surfaces of biomaterials to enhance their biocompatibility and functionality.
Comparison with Similar Compounds
N-Hydroxysuccinimide Esters: These esters, in general, share similar reactivity and applications. Examples include esters of other carboxylic acids like acetic acid N-hydroxysuccinimide ester.
Sulfo-N-Hydroxysuccinimide Esters: These esters contain a sulfonate group, making them more water-soluble and suitable for aqueous reactions.
Uniqueness: Butyric acid N-hydroxysuccinimide ester is unique due to the presence of the butyric acid moiety, which can impart specific properties to the conjugated biomolecules, such as increased hydrophobicity or altered biological activity. This makes it particularly useful in applications where these properties are desired.
Properties
IUPAC Name |
(2,5-dioxopyrrolidin-1-yl) butanoate | |
---|---|---|
Details | Computed by LexiChem 2.6.6 (PubChem release 2019.06.18) | |
Source | PubChem | |
URL | https://pubchem.ncbi.nlm.nih.gov | |
Description | Data deposited in or computed by PubChem | |
InChI |
InChI=1S/C8H11NO4/c1-2-3-8(12)13-9-6(10)4-5-7(9)11/h2-5H2,1H3 | |
Details | Computed by InChI 1.0.5 (PubChem release 2019.06.18) | |
Source | PubChem | |
URL | https://pubchem.ncbi.nlm.nih.gov | |
Description | Data deposited in or computed by PubChem | |
InChI Key |
DYMYLBQTHCJHOQ-UHFFFAOYSA-N | |
Details | Computed by InChI 1.0.5 (PubChem release 2019.06.18) | |
Source | PubChem | |
URL | https://pubchem.ncbi.nlm.nih.gov | |
Description | Data deposited in or computed by PubChem | |
Canonical SMILES |
CCCC(=O)ON1C(=O)CCC1=O | |
Details | Computed by OEChem 2.1.5 (PubChem release 2019.06.18) | |
Source | PubChem | |
URL | https://pubchem.ncbi.nlm.nih.gov | |
Description | Data deposited in or computed by PubChem | |
Molecular Formula |
C8H11NO4 | |
Details | Computed by PubChem 2.1 (PubChem release 2019.06.18) | |
Source | PubChem | |
URL | https://pubchem.ncbi.nlm.nih.gov | |
Description | Data deposited in or computed by PubChem | |
Molecular Weight |
185.18 g/mol | |
Details | Computed by PubChem 2.1 (PubChem release 2021.05.07) | |
Source | PubChem | |
URL | https://pubchem.ncbi.nlm.nih.gov | |
Description | Data deposited in or computed by PubChem | |
Synthesis routes and methods
Procedure details
Retrosynthesis Analysis
AI-Powered Synthesis Planning: Our tool employs the Template_relevance Pistachio, Template_relevance Bkms_metabolic, Template_relevance Pistachio_ringbreaker, Template_relevance Reaxys, Template_relevance Reaxys_biocatalysis model, leveraging a vast database of chemical reactions to predict feasible synthetic routes.
One-Step Synthesis Focus: Specifically designed for one-step synthesis, it provides concise and direct routes for your target compounds, streamlining the synthesis process.
Accurate Predictions: Utilizing the extensive PISTACHIO, BKMS_METABOLIC, PISTACHIO_RINGBREAKER, REAXYS, REAXYS_BIOCATALYSIS database, our tool offers high-accuracy predictions, reflecting the latest in chemical research and data.
Strategy Settings
Precursor scoring | Relevance Heuristic |
---|---|
Min. plausibility | 0.01 |
Model | Template_relevance |
Template Set | Pistachio/Bkms_metabolic/Pistachio_ringbreaker/Reaxys/Reaxys_biocatalysis |
Top-N result to add to graph | 6 |
Feasible Synthetic Routes
Disclaimer and Information on In-Vitro Research Products
Please be aware that all articles and product information presented on BenchChem are intended solely for informational purposes. The products available for purchase on BenchChem are specifically designed for in-vitro studies, which are conducted outside of living organisms. In-vitro studies, derived from the Latin term "in glass," involve experiments performed in controlled laboratory settings using cells or tissues. It is important to note that these products are not categorized as medicines or drugs, and they have not received approval from the FDA for the prevention, treatment, or cure of any medical condition, ailment, or disease. We must emphasize that any form of bodily introduction of these products into humans or animals is strictly prohibited by law. It is essential to adhere to these guidelines to ensure compliance with legal and ethical standards in research and experimentation.