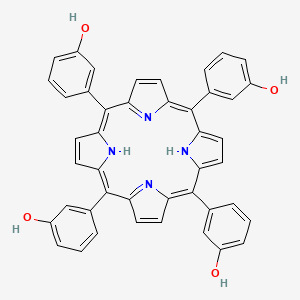
Tetra(3-hydroxyphenyl)porphyrin
Overview
Description
Tetra(3-hydroxyphenyl)porphyrin is a synthetic porphyrin derivative characterized by the presence of four hydroxyphenyl groups attached to the meso positions of the porphyrin ring. Porphyrins are macrocyclic compounds that play crucial roles in various biological processes, such as oxygen transport and photosynthesis.
Mechanism of Action
Tetra(3-hydroxyphenyl)porphyrin, also known as 3,3’,3’‘,3’‘’-(21H,23H-Porphine-5,10,15,20-tetrayl)tetrakisphenol, is a compound that has attracted significant interest due to its potential applications in photodynamic therapy .
Target of Action
The primary targets of this compound are cancer cells . The compound has superior tumor-localizing properties, which makes it an effective photosensitizer .
Mode of Action
This compound acts as a photosensitizing agent . It enters cancer cells and is activated via light to produce reactive species which destroy the cell . The compound is excited from ground state to the first excited singlet state by the application of 652 nm light . It then undergoes intersystem crossing to an excited triplet state which is longer lived and able to interact with surrounding molecules .
Pharmacokinetics
The pharmacokinetics of this compound involve its distribution between normal and cancer tissue . The compound shows high localization in cancer tissue . The development of supramolecular platforms has improved the compound’s pharmacokinetics and tumor-homing .
Result of Action
The result of the compound’s action is the destruction of cancer cells . The compound has a high tumor-necrosis/normal-tissue damage ratio when used as a photosensitizer . This means that it effectively destroys cancer cells while minimizing damage to normal tissue .
Action Environment
The action of this compound is influenced by environmental factors. For instance, the compound shows high toxicity in the dark . This suggests that the compound’s action, efficacy, and stability are influenced by light conditions .
Biochemical Analysis
Biochemical Properties
Tetra(3-hydroxyphenyl)porphyrin plays a crucial role in biochemical reactions, particularly in photodynamic therapy. It acts as a photosensitizer, absorbing light and transferring energy to molecular oxygen to produce reactive oxygen species, which can induce cell death. This compound interacts with various biomolecules, including enzymes and proteins. For instance, it has been shown to interact with cytochrome P450 enzymes, influencing their catalytic activity . Additionally, this compound can bind to albumin, facilitating its transport in the bloodstream .
Cellular Effects
This compound exerts significant effects on various cell types and cellular processes. In cancer cells, it has been observed to localize within intracellular membranes and lysosomes, leading to the induction of apoptosis upon light activation . This compound influences cell signaling pathways, such as the mitochondrial apoptotic pathway, by generating reactive oxygen species that cause mitochondrial damage and release of cytochrome c . Furthermore, this compound can alter gene expression, upregulating pro-apoptotic genes and downregulating anti-apoptotic genes .
Molecular Mechanism
The molecular mechanism of this compound involves its ability to generate reactive oxygen species upon light activation. This process begins with the absorption of light by the porphyrin ring, leading to an excited singlet state. The energy is then transferred to molecular oxygen, producing singlet oxygen and other reactive oxygen species . These reactive species can cause oxidative damage to cellular components, including lipids, proteins, and DNA . This compound can also bind to specific biomolecules, such as cytochrome P450 enzymes, modulating their activity .
Temporal Effects in Laboratory Settings
In laboratory settings, the effects of this compound can change over time. The compound is relatively stable under normal conditions but can degrade upon prolonged exposure to light and oxygen . In vitro studies have shown that the photodynamic effects of this compound can persist for several hours after light activation, leading to sustained cellular damage . Long-term studies in vivo have indicated that repeated administration of this compound can result in cumulative effects, including enhanced tumor necrosis and reduced normal tissue damage .
Dosage Effects in Animal Models
The effects of this compound vary with different dosages in animal models. At low doses, the compound can selectively target tumor cells with minimal effects on normal tissues . At higher doses, it can cause significant toxicity, including skin photosensitivity and damage to normal tissues . Threshold effects have been observed, where a minimum dose is required to achieve therapeutic efficacy, while doses above this threshold can lead to adverse effects .
Metabolic Pathways
This compound is involved in several metabolic pathways. It can be metabolized by cytochrome P450 enzymes, leading to the formation of various metabolites . These metabolites can further interact with other enzymes and cofactors, influencing metabolic flux and altering metabolite levels . The compound’s metabolism can also affect its photodynamic activity, with certain metabolites exhibiting reduced or enhanced photosensitizing properties .
Transport and Distribution
Within cells and tissues, this compound is transported and distributed through interactions with transporters and binding proteins. Albumin plays a significant role in its transport in the bloodstream, facilitating its delivery to target tissues . The compound can also interact with cellular transporters, influencing its uptake and distribution within cells . Its localization and accumulation in specific tissues can be affected by factors such as tissue perfusion and the presence of specific receptors .
Subcellular Localization
This compound exhibits specific subcellular localization, which can affect its activity and function. It has been observed to localize within intracellular membranes, lysosomes, and mitochondria . This localization is influenced by targeting signals and post-translational modifications that direct the compound to specific compartments . The subcellular localization of this compound is crucial for its photodynamic activity, as it determines the sites of reactive oxygen species generation and subsequent cellular damage .
Preparation Methods
Synthetic Routes and Reaction Conditions: The synthesis of tetra(3-hydroxyphenyl)porphyrin typically involves the Adler method, which entails the condensation of pyrrole with 3-hydroxybenzaldehyde in the presence of an acid catalyst, such as propionic acid. The reaction is carried out under reflux conditions, and the resulting porphyrin is purified through column chromatography .
Industrial Production Methods: Industrial production of this compound follows similar synthetic routes but often employs scalable techniques such as microwave-assisted synthesis or mechanochemistry to enhance yield and reduce reaction times. These methods are designed to be more sustainable and environmentally friendly .
Chemical Reactions Analysis
Types of Reactions: Tetra(3-hydroxyphenyl)porphyrin undergoes various chemical reactions, including:
Oxidation: The hydroxy groups can be oxidized to quinones under specific conditions.
Reduction: The porphyrin ring can be reduced to form chlorins or bacteriochlorins.
Substitution: The hydroxy groups can participate in nucleophilic substitution reactions to introduce different functional groups.
Common Reagents and Conditions:
Oxidation: Reagents such as potassium permanganate or chromium trioxide are commonly used.
Reduction: Sodium borohydride or lithium aluminum hydride are typical reducing agents.
Substitution: Reagents like alkyl halides or acyl chlorides are used for nucleophilic substitution.
Major Products Formed:
Oxidation: Quinone derivatives.
Reduction: Chlorins or bacteriochlorins.
Substitution: Various functionalized porphyrins depending on the substituents introduced.
Scientific Research Applications
Tetra(3-hydroxyphenyl)porphyrin has a wide range of applications in scientific research:
Chemistry: Used as a catalyst in organic synthesis and photocatalysis.
Biology: Employed in studies of enzyme mimetics and as a model compound for heme proteins.
Medicine: Utilized in photodynamic therapy for cancer treatment due to its ability to generate singlet oxygen upon light activation.
Industry: Applied in the development of sensors, solar cells, and advanced materials.
Comparison with Similar Compounds
Tetra(4-hydroxyphenyl)porphyrin: Similar structure but with hydroxy groups in the para position.
Tetra(2-hydroxyphenyl)porphyrin: Hydroxy groups in the ortho position.
Tetra(3,5-dihydroxyphenyl)porphyrin: Contains additional hydroxy groups.
Uniqueness: Tetra(3-hydroxyphenyl)porphyrin is unique due to the meta positioning of the hydroxy groups, which influences its photophysical properties and reactivity. This positioning enhances its ability to generate singlet oxygen and improves its solubility and cellular uptake compared to its ortho and para counterparts .
Properties
IUPAC Name |
3-[10,15,20-tris(3-hydroxyphenyl)-21,23-dihydroporphyrin-5-yl]phenol | |
---|---|---|
Details | Computed by Lexichem TK 2.7.0 (PubChem release 2021.05.07) | |
Source | PubChem | |
URL | https://pubchem.ncbi.nlm.nih.gov | |
Description | Data deposited in or computed by PubChem | |
InChI |
InChI=1S/C44H30N4O4/c49-29-9-1-5-25(21-29)41-33-13-15-35(45-33)42(26-6-2-10-30(50)22-26)37-17-19-39(47-37)44(28-8-4-12-32(52)24-28)40-20-18-38(48-40)43(36-16-14-34(41)46-36)27-7-3-11-31(51)23-27/h1-24,45,48-52H | |
Details | Computed by InChI 1.0.6 (PubChem release 2021.05.07) | |
Source | PubChem | |
URL | https://pubchem.ncbi.nlm.nih.gov | |
Description | Data deposited in or computed by PubChem | |
InChI Key |
ZUELZXZJXUXJCH-UHFFFAOYSA-N | |
Details | Computed by InChI 1.0.6 (PubChem release 2021.05.07) | |
Source | PubChem | |
URL | https://pubchem.ncbi.nlm.nih.gov | |
Description | Data deposited in or computed by PubChem | |
Canonical SMILES |
C1=CC(=CC(=C1)O)C2=C3C=CC(=C(C4=NC(=C(C5=CC=C(N5)C(=C6C=CC2=N6)C7=CC(=CC=C7)O)C8=CC(=CC=C8)O)C=C4)C9=CC(=CC=C9)O)N3 | |
Details | Computed by OEChem 2.3.0 (PubChem release 2021.05.07) | |
Source | PubChem | |
URL | https://pubchem.ncbi.nlm.nih.gov | |
Description | Data deposited in or computed by PubChem | |
Molecular Formula |
C44H30N4O4 | |
Details | Computed by PubChem 2.1 (PubChem release 2021.05.07) | |
Source | PubChem | |
URL | https://pubchem.ncbi.nlm.nih.gov | |
Description | Data deposited in or computed by PubChem | |
DSSTOX Substance ID |
DTXSID401025582 | |
Record name | 5,10,15,20-Tetrakis(3-hydroxyphenyl)porphyrin | |
Source | EPA DSSTox | |
URL | https://comptox.epa.gov/dashboard/DTXSID401025582 | |
Description | DSSTox provides a high quality public chemistry resource for supporting improved predictive toxicology. | |
Molecular Weight |
678.7 g/mol | |
Details | Computed by PubChem 2.1 (PubChem release 2021.05.07) | |
Source | PubChem | |
URL | https://pubchem.ncbi.nlm.nih.gov | |
Description | Data deposited in or computed by PubChem | |
CAS No. |
22112-79-4 | |
Record name | 5,10,15,20-Tetra(3-hydroxyphenyl)porphyrin | |
Source | ChemIDplus | |
URL | https://pubchem.ncbi.nlm.nih.gov/substance/?source=chemidplus&sourceid=0022112794 | |
Description | ChemIDplus is a free, web search system that provides access to the structure and nomenclature authority files used for the identification of chemical substances cited in National Library of Medicine (NLM) databases, including the TOXNET system. | |
Record name | 5,10,15,20-Tetrakis(3-hydroxyphenyl)porphyrin | |
Source | EPA DSSTox | |
URL | https://comptox.epa.gov/dashboard/DTXSID401025582 | |
Description | DSSTox provides a high quality public chemistry resource for supporting improved predictive toxicology. | |
Synthesis routes and methods
Procedure details
Disclaimer and Information on In-Vitro Research Products
Please be aware that all articles and product information presented on BenchChem are intended solely for informational purposes. The products available for purchase on BenchChem are specifically designed for in-vitro studies, which are conducted outside of living organisms. In-vitro studies, derived from the Latin term "in glass," involve experiments performed in controlled laboratory settings using cells or tissues. It is important to note that these products are not categorized as medicines or drugs, and they have not received approval from the FDA for the prevention, treatment, or cure of any medical condition, ailment, or disease. We must emphasize that any form of bodily introduction of these products into humans or animals is strictly prohibited by law. It is essential to adhere to these guidelines to ensure compliance with legal and ethical standards in research and experimentation.