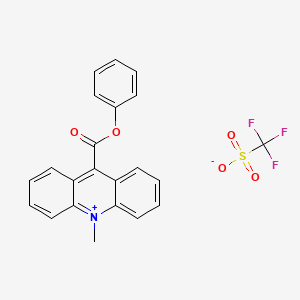
10-Methyl-9-(phenoxycarbonyl)-acridinium,1,1,1-trifluoromethanesulfonate
Overview
Description
10-methyl-9-(phenoxycarbonyl) Acridinium (trifluoromethylsulfonate): is an acridinium ester known for its chemiluminescent properties. Upon oxidation with hydrogen peroxide, persulfates, and other oxidants under alkaline conditions, it produces fluorescent 10-methyl-9-acridone . This compound is widely used in chemiluminescence assays, enzyme, antigen, antibody, and hormone immunoassays, as well as in the detection of oxidants in environmental, biological, and pharmaceutical samples .
Mechanism of Action
Target of Action
The primary target of 10-Methyl-9-(phenoxycarbonyl)-acridinium,1,1,1-trifluoromethanesulfonate is the electronically excited 10-methyl-9-acridinone . This compound is a chemiluminescent acridinium ester that produces fluorescent 10-methyl-9-acridone upon oxidation .
Mode of Action
In the reaction with OOH(-) in basic aqueous media, the cations of the compounds investigated were converted to electronically excited 10-methyl-9-acridinone . The chemiluminescence efficiency is the result of competition of the electrophilic center at C(9) between nucleophilic substitution by OOH(-) or OH(-) and the ability of the intermediates thus formed to decompose to electronically excited 10-methyl-9-acridinone .
Biochemical Pathways
The compound operates within the biochemical pathway of chemiluminescence, specifically in the oxidation of acridinium esters . The process is accompanied by the relatively effective emission of light, reaching quantum yields of approximately 2% and lying within the blue range of the spectrum .
Pharmacokinetics
It’s known that the compound is synthesized, purified, and identified . More research would be needed to fully understand its ADME properties and their impact on bioavailability.
Result of Action
The result of the action of this compound is the production of chemiluminescence . This is a process that emits light as a result of a chemical reaction. In this case, the reaction involves the oxidation of the compound, resulting in the emission of light .
Action Environment
The action of this compound is influenced by the environment in which it is present. The chemiluminescent properties of the compound were investigated in aliphatic alcohols, acetonitrile, and dimethyl sulfoxide in the presence of hydrogen peroxide and different bases . The values of decay rate constants and relative efficiencies depend linearly on polarity and acid-base properties of solvents as well as on concentration of bases .
Biochemical Analysis
Biochemical Properties
10-Methyl-9-(phenoxycarbonyl)-acridinium,1,1,1-trifluoromethanesulfonate interacts with various enzymes, proteins, and other biomolecules in biochemical reactions . It produces fluorescent 10-methyl-9-acridone upon oxidation with hydrogen peroxide, persulfate, and other oxidants under alkaline conditions . The nature of these interactions is primarily through the process of oxidation.
Molecular Mechanism
The molecular mechanism of action of this compound involves its oxidation to produce fluorescent 10-methyl-9-acridone This process may involve binding interactions with biomolecules, enzyme inhibition or activation, and changes in gene expression
Preparation Methods
Synthetic Routes and Reaction Conditions: The synthesis of 10-methyl-9-(phenoxycarbonyl) Acridinium (trifluoromethylsulfonate) involves the reaction of 10-methylacridone with phenyl chloroformate in the presence of a base, followed by the addition of trifluoromethanesulfonic acid . The reaction typically occurs under mild conditions, and the product is purified through recrystallization.
Industrial Production Methods: Industrial production methods for this compound are not extensively documented. the synthesis generally follows similar routes as laboratory methods, with optimizations for scale, yield, and purity .
Chemical Reactions Analysis
Types of Reactions:
Substitution: The phenoxycarbonyl group can be substituted with other functional groups under appropriate conditions.
Common Reagents and Conditions:
Oxidation: Hydrogen peroxide, persulfates, and other oxidants in alkaline conditions.
Substitution: Various nucleophiles can be used for substitution reactions.
Major Products:
Scientific Research Applications
Comparison with Similar Compounds
10-Methyl-9-(phenoxycarbonyl)acridinium fluorosulfonate: Another acridinium ester with similar chemiluminescent properties.
9-Mesityl-2,7-dimethyl-10-phenylacridinium tetrafluoroborate: A related compound used in chemiluminescent assays.
9-Mesityl-10-methylacridinium tetrafluoroborate: Another acridinium derivative with chemiluminescent properties.
Uniqueness: 10-methyl-9-(phenoxycarbonyl) Acridinium (trifluoromethylsulfonate) is unique due to its high chemiluminescent efficiency under neutral conditions, making it suitable for a wide range of applications in both research and industry .
Properties
IUPAC Name |
phenyl 10-methylacridin-10-ium-9-carboxylate;trifluoromethanesulfonate | |
---|---|---|
Details | Computed by Lexichem TK 2.7.0 (PubChem release 2021.05.07) | |
Source | PubChem | |
URL | https://pubchem.ncbi.nlm.nih.gov | |
Description | Data deposited in or computed by PubChem | |
InChI |
InChI=1S/C21H16NO2.CHF3O3S/c1-22-18-13-7-5-11-16(18)20(17-12-6-8-14-19(17)22)21(23)24-15-9-3-2-4-10-15;2-1(3,4)8(5,6)7/h2-14H,1H3;(H,5,6,7)/q+1;/p-1 | |
Details | Computed by InChI 1.0.6 (PubChem release 2021.05.07) | |
Source | PubChem | |
URL | https://pubchem.ncbi.nlm.nih.gov | |
Description | Data deposited in or computed by PubChem | |
InChI Key |
RPYQDSABWVKCEJ-UHFFFAOYSA-M | |
Details | Computed by InChI 1.0.6 (PubChem release 2021.05.07) | |
Source | PubChem | |
URL | https://pubchem.ncbi.nlm.nih.gov | |
Description | Data deposited in or computed by PubChem | |
Canonical SMILES |
C[N+]1=C2C=CC=CC2=C(C3=CC=CC=C31)C(=O)OC4=CC=CC=C4.C(F)(F)(F)S(=O)(=O)[O-] | |
Details | Computed by OEChem 2.3.0 (PubChem release 2021.05.07) | |
Source | PubChem | |
URL | https://pubchem.ncbi.nlm.nih.gov | |
Description | Data deposited in or computed by PubChem | |
Molecular Formula |
C22H16F3NO5S | |
Details | Computed by PubChem 2.1 (PubChem release 2021.05.07) | |
Source | PubChem | |
URL | https://pubchem.ncbi.nlm.nih.gov | |
Description | Data deposited in or computed by PubChem | |
Molecular Weight |
463.4 g/mol | |
Details | Computed by PubChem 2.1 (PubChem release 2021.05.07) | |
Source | PubChem | |
URL | https://pubchem.ncbi.nlm.nih.gov | |
Description | Data deposited in or computed by PubChem | |
Synthesis routes and methods I
Procedure details
Synthesis routes and methods II
Procedure details
Retrosynthesis Analysis
AI-Powered Synthesis Planning: Our tool employs the Template_relevance Pistachio, Template_relevance Bkms_metabolic, Template_relevance Pistachio_ringbreaker, Template_relevance Reaxys, Template_relevance Reaxys_biocatalysis model, leveraging a vast database of chemical reactions to predict feasible synthetic routes.
One-Step Synthesis Focus: Specifically designed for one-step synthesis, it provides concise and direct routes for your target compounds, streamlining the synthesis process.
Accurate Predictions: Utilizing the extensive PISTACHIO, BKMS_METABOLIC, PISTACHIO_RINGBREAKER, REAXYS, REAXYS_BIOCATALYSIS database, our tool offers high-accuracy predictions, reflecting the latest in chemical research and data.
Strategy Settings
Precursor scoring | Relevance Heuristic |
---|---|
Min. plausibility | 0.01 |
Model | Template_relevance |
Template Set | Pistachio/Bkms_metabolic/Pistachio_ringbreaker/Reaxys/Reaxys_biocatalysis |
Top-N result to add to graph | 6 |
Feasible Synthetic Routes
Disclaimer and Information on In-Vitro Research Products
Please be aware that all articles and product information presented on BenchChem are intended solely for informational purposes. The products available for purchase on BenchChem are specifically designed for in-vitro studies, which are conducted outside of living organisms. In-vitro studies, derived from the Latin term "in glass," involve experiments performed in controlled laboratory settings using cells or tissues. It is important to note that these products are not categorized as medicines or drugs, and they have not received approval from the FDA for the prevention, treatment, or cure of any medical condition, ailment, or disease. We must emphasize that any form of bodily introduction of these products into humans or animals is strictly prohibited by law. It is essential to adhere to these guidelines to ensure compliance with legal and ethical standards in research and experimentation.