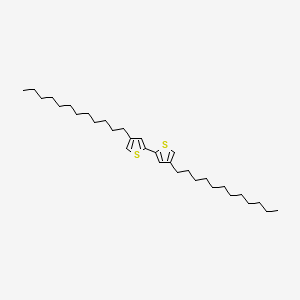
4,4'-Didodecyl-2,2'-bithiophene
Overview
Description
4,4'-Didodecyl-2,2'-bithiophene (DDD-BT) is a symmetrically alkyl-substituted bithiophene derivative synthesized via coupling of 3-dodecylthiophene monomers . Its structure features two dodecyl chains at the 4,4' positions of the bithiophene core, enhancing solubility and enabling molecular self-assembly in thin-film applications. It is also a precursor to brominated derivatives (e.g., 5,5'-dibromo-DDD-BT), widely used in Stille polycondensation reactions to synthesize high-performance polymers such as PBTTT (poly(bithiophene-thienothiophene)) with charge carrier mobilities of 0.2–0.6 cm² V⁻¹ s⁻¹ . Applications span organic photovoltaics (OPV), field-effect transistors (OFETs), and biosensors .
Preparation Methods
Synthetic Routes and Reaction Conditions: The synthesis of 4,4’-Didodecyl-2,2’-bithiophene typically involves the bromination of 4,4’-didodecyl-2,2’-bithiophene to form 5,5’-dibromo-4,4’-didodecyl-2,2’-bithiophene. This intermediate can then undergo various coupling reactions to form the desired product. The reaction conditions often include the use of palladium catalysts and specific solvents to facilitate the coupling reactions .
Industrial Production Methods: In industrial settings, the production of 4,4’-Didodecyl-2,2’-bithiophene involves large-scale bromination and coupling reactions. The use of high-purity reagents and optimized reaction conditions ensures the efficient production of the compound with minimal impurities .
Chemical Reactions Analysis
Types of Reactions: 4,4’-Didodecyl-2,2’-bithiophene undergoes various chemical reactions, including:
Oxidation: The compound can be oxidized to form sulfoxides or sulfones.
Reduction: Reduction reactions can convert the thiophene rings to dihydrothiophenes.
Substitution: Halogenation and other substitution reactions can introduce different functional groups onto the thiophene rings.
Common Reagents and Conditions:
Oxidation: Common oxidizing agents include hydrogen peroxide and m-chloroperbenzoic acid.
Reduction: Reducing agents such as lithium aluminum hydride and sodium borohydride are used.
Substitution: Halogenation reactions often use bromine or chlorine in the presence of catalysts.
Major Products: The major products formed from these reactions include various substituted thiophenes, sulfoxides, and sulfones, depending on the specific reaction conditions and reagents used .
Scientific Research Applications
4,4’-Didodecyl-2,2’-bithiophene has a wide range of applications in scientific research:
Biology: The compound’s derivatives are studied for their potential biological activities and interactions with biomolecules.
Medicine: Research is ongoing to explore the potential therapeutic applications of thiophene derivatives in drug development.
Mechanism of Action
The mechanism of action of 4,4’-Didodecyl-2,2’-bithiophene in organic electronics involves its ability to facilitate charge transport. The dodecyl side chains enhance the solubility and film-forming properties of the compound, allowing for better intermolecular packing and π–π stacking interactions. These properties contribute to the high charge mobility observed in semiconductive polymers derived from 4,4’-Didodecyl-2,2’-bithiophene .
Comparison with Similar Compounds
Comparison with Structural Analogs
Substituent Effects on Solubility and Molecular Packing
4,4'-Bis(2-Methyl-3-Butyn-2-ol)-2,2'-Bithiophene (4,4'-bBT)
- Structure : Bulky, polar 2-methyl-3-butyn-2-ol substituents at 4,4' positions.
- Properties : Forms electrochemically polymerized films with high porosity and stability. Used in glucose biosensors, outperforming 2,2'-bithiophene (2,2'-BT) due to lower detection limits (0.5 μM vs. 1.2 μM) and faster response times .
- Key Difference: Polar substituents enhance film adhesion and mediator (p-benzoquinone) interaction, improving biosensor performance.
4,4'-Dihexyl-2,2'-Bithiophene (Tail-to-Tail Coupling)
- Structure : Linear hexyl chains at 4,4' positions.
- Properties : Promotes edge-on molecular packing in copolymers, improving charge transport in benzotrithiophene (BTT)-based polymers. Comparatively, 3,3'-dihexyl-2,2'-bithiophene (head-to-head coupling) induces disordered packing .
3,3′-Difluoro-4,4′-Dihexadecyl-2,2′-Bithiophene
- Structure : Fluorination at 3,3' positions and hexadecyl chains at 4,4'.
- Properties : Fluorine’s electron-withdrawing effect enhances backbone rigidity and aggregation, leading to higher crystallinity and charge mobility in fluorinated PBTTT analogs .
Electronic Properties and Charge Transport
- BTDCA Copolymers : Incorporation of [2,2'-bithiophene]-4,4'-dicarboxamide (BTDCA) reduces HOMO levels by 0.3 eV vs. polythiophenes, increasing open-circuit voltage (Voc) in solar cells .
Application-Specific Performance
- Biosensors : 4,4'-bBT films outperform 2,2'-BT in glucose detection due to optimized film morphology .
- OPVs : BTDCA-based polymers achieve power conversion efficiencies (PCE) >8% via HOMO engineering .
- OFETs : PBTTT-C12 (from DDD-BT) shows ambipolar transport, while fluorinated analogs exhibit unipolar p-type behavior .
Biological Activity
4,4'-Didodecyl-2,2'-bithiophene (DDTBT) is a compound belonging to the class of bithiophene derivatives, which are of significant interest in organic electronics and materials science due to their unique electronic properties. Recent studies have begun to explore the biological activity of DDTBT, revealing potential applications in biomedical fields, particularly in drug delivery and as therapeutic agents.
Chemical Structure and Properties
The chemical structure of this compound consists of two thiophene rings connected by a single bond with dodecyl chains at the 4-position of each thiophene. This structure contributes to its solubility and stability in organic solvents.
Research indicates that DDTBT may interact with biological systems through several mechanisms:
- Antimicrobial Activity : DDTBT exhibits significant antimicrobial properties against various bacterial strains. Its mechanism involves disrupting bacterial cell membranes, leading to cell lysis.
- Anticancer Properties : Preliminary studies suggest that DDTBT can inhibit cancer cell proliferation. The compound may induce apoptosis in cancer cells by activating specific signaling pathways.
- Biocompatibility : DDTBT shows high biocompatibility, making it suitable for biomedical applications such as drug delivery systems.
Antimicrobial Activity
A study evaluated the antimicrobial efficacy of DDTBT against Gram-positive and Gram-negative bacteria. The results demonstrated a minimum inhibitory concentration (MIC) of 50 µg/mL for Staphylococcus aureus and 75 µg/mL for Escherichia coli.
Bacterial Strain | Minimum Inhibitory Concentration (MIC) |
---|---|
Staphylococcus aureus | 50 µg/mL |
Escherichia coli | 75 µg/mL |
Anticancer Activity
In vitro assays revealed that DDTBT significantly reduced the viability of human breast cancer cells (MCF-7) at concentrations ranging from 10 to 100 µM. The compound induced apoptosis as evidenced by increased caspase-3 activity.
Concentration (µM) | Cell Viability (%) | Caspase-3 Activity (Relative Units) |
---|---|---|
10 | 80 | 1.5 |
50 | 50 | 3.0 |
100 | 30 | 5.5 |
Case Studies
- Case Study on Drug Delivery : A formulation study investigated the use of DDTBT as a carrier for anticancer drugs. Results indicated enhanced solubility and controlled release properties when combined with doxorubicin.
- Case Study on Biocompatibility : An animal study assessed the biocompatibility of DDTBT when implanted subcutaneously. Histological analysis showed minimal inflammatory response, indicating good biocompatibility.
Q & A
Q. Basic: What are the established synthesis routes for 4,4'-didodecyl-2,2'-bithiophene, and how is its purity validated?
The compound is synthesized via cross-coupling reactions. A common method involves Stille coupling of 3-dodecylthiophene derivatives using Pd catalysts (e.g., Pd₂(dba)₃ with P(o-tolyl)₃ in chlorobenzene under microwave heating) . Post-synthesis, purification is achieved through Soxhlet extraction with solvents like acetone, hexane, and chloroform, followed by precipitation in methanol . Purity is validated via:
- ¹H/¹³C NMR : Peaks for dodecyl chains (δ ~0.88 ppm for terminal CH₃, δ ~29–32 ppm for CH₂ carbons) and thiophene protons (δ ~6.77 ppm) .
- Gel Permeation Chromatography (GPC) : Determines molecular weight (Mn ~21–34 kDa) and polydispersity (Đ ~1.58) .
- Thermogravimetric Analysis (TGA) : Confirms thermal stability up to ~300°C under nitrogen .
Q. Basic: How is this compound integrated into semiconducting polymers?
The monomer is polymerized via:
- Stille Coupling : Reacted with stannylated comonomers (e.g., 2,5-bis(trimethylstannyl)thieno[3,2-b]thiophene) using Pd catalysts .
- Direct Arylation : Phosphine-free Pd-catalyzed coupling with electron-deficient acceptors (e.g., thiophene–phenylene–thiophene bislactam) to form donor-acceptor copolymers .
- Polycondensation : Hydrazine acetate in chloroform under reflux forms azine-linked polymers (e.g., PDDBTA) .
Key parameters include reaction temperature (140–180°C for microwave-assisted methods) and stoichiometric control to minimize unreacted termini .
Q. Advanced: How do morphological and electronic properties influence charge transport in OTFTs?
- Morphology : Atomic force microscopy (AFM) reveals film crystallinity, critical for mobility. High-temperature annealing (e.g., 110°C) enhances molecular ordering .
- SCLC Analysis : Hole mobility (μ) is calculated using space-charge-limited current models:
where (relative permittivity) and film thickness () are measured via ellipsometry . - OTFT Performance : Bottom-gate configurations yield mobilities of 0.2–0.6 cm² V⁻¹ s⁻¹. Discrepancies arise from solvent choice (chloroform vs. trichlorobenzene) and substrate treatments .
Q. Advanced: How to resolve contradictions in reported charge mobility values?
Discrepancies stem from:
- Synthetic Conditions : Microwave vs. conventional heating alters polymer chain alignment .
- Doping Ratios : Incorporating electron-deficient units (e.g., 5,5'-dibromo derivatives) reduces bandgap but may introduce traps .
- Characterization Methods : SCLC vs. field-effect measurements differ in bias regimes. Cross-validate using cyclic voltammetry (HOMO/LUMO levels) and grazing-incidence XRD (crystallinity) .
Q. Advanced: What strategies optimize electronic properties for NIR-II photothermal therapy?
- Bandgap Engineering : Co-polymerizing with weak electron donors (e.g., 5,5'-dibromo-4,4'-didodecyl-2,2'-bithiophene) redshifts absorption via "strong-strong" coupling .
- Doping Gradients : Adjusting donor-acceptor ratios in OSPs (e.g., OSP 21, 11, 12) tunes NIR-II brightness and thermal conversion efficiency .
- Spectroscopic Validation : UV-vis-NIR and photoluminescence quenching quantify exciton dissociation efficiency .
Q. Methodological: How to handle air-sensitive intermediates during synthesis?
- Schlenk Techniques : Use argon/vacuum cycles for monomer purification .
- Dry Solvents : Reflux chloroform over CaH₂ or molecular sieves .
- Termination Agents : Add 2-thiophenecarboxaldehyde to quench residual hydrazine .
Q. Advanced: What thermal processing conditions ensure stability in device fabrication?
- TGA-Guided Annealing : Degradation onset at ~300°C suggests safe processing below 250°C .
- Solution Shearing : Optimize film uniformity by adjusting solvent boiling points (e.g., 1,2,4-trichlorobenzene at 110°C) .
Q. Advanced: Why do optical properties vary across polymer batches?
- Regiochemistry : β-substitution in thiophene derivatives affects conjugation length .
- Aggregation States : UV-vis spectra (e.g., λₘₐₓ ~450–600 nm) shift with solvent polarity (THF vs. chloroform) .
- PL Quenching : Use Horiba PTI fluorimeters to correlate quenching efficiency with exciton diffusion lengths .
Properties
IUPAC Name |
4-dodecyl-2-(4-dodecylthiophen-2-yl)thiophene | |
---|---|---|
Details | Computed by Lexichem TK 2.7.0 (PubChem release 2021.05.07) | |
Source | PubChem | |
URL | https://pubchem.ncbi.nlm.nih.gov | |
Description | Data deposited in or computed by PubChem | |
InChI |
InChI=1S/C32H54S2/c1-3-5-7-9-11-13-15-17-19-21-23-29-25-31(33-27-29)32-26-30(28-34-32)24-22-20-18-16-14-12-10-8-6-4-2/h25-28H,3-24H2,1-2H3 | |
Details | Computed by InChI 1.0.6 (PubChem release 2021.05.07) | |
Source | PubChem | |
URL | https://pubchem.ncbi.nlm.nih.gov | |
Description | Data deposited in or computed by PubChem | |
InChI Key |
DWWZELSEBSRUMM-UHFFFAOYSA-N | |
Details | Computed by InChI 1.0.6 (PubChem release 2021.05.07) | |
Source | PubChem | |
URL | https://pubchem.ncbi.nlm.nih.gov | |
Description | Data deposited in or computed by PubChem | |
Canonical SMILES |
CCCCCCCCCCCCC1=CSC(=C1)C2=CC(=CS2)CCCCCCCCCCCC | |
Details | Computed by OEChem 2.3.0 (PubChem release 2021.05.07) | |
Source | PubChem | |
URL | https://pubchem.ncbi.nlm.nih.gov | |
Description | Data deposited in or computed by PubChem | |
Molecular Formula |
C32H54S2 | |
Details | Computed by PubChem 2.1 (PubChem release 2021.05.07) | |
Source | PubChem | |
URL | https://pubchem.ncbi.nlm.nih.gov | |
Description | Data deposited in or computed by PubChem | |
Molecular Weight |
502.9 g/mol | |
Details | Computed by PubChem 2.1 (PubChem release 2021.05.07) | |
Source | PubChem | |
URL | https://pubchem.ncbi.nlm.nih.gov | |
Description | Data deposited in or computed by PubChem | |
Synthesis routes and methods
Procedure details
Disclaimer and Information on In-Vitro Research Products
Please be aware that all articles and product information presented on BenchChem are intended solely for informational purposes. The products available for purchase on BenchChem are specifically designed for in-vitro studies, which are conducted outside of living organisms. In-vitro studies, derived from the Latin term "in glass," involve experiments performed in controlled laboratory settings using cells or tissues. It is important to note that these products are not categorized as medicines or drugs, and they have not received approval from the FDA for the prevention, treatment, or cure of any medical condition, ailment, or disease. We must emphasize that any form of bodily introduction of these products into humans or animals is strictly prohibited by law. It is essential to adhere to these guidelines to ensure compliance with legal and ethical standards in research and experimentation.