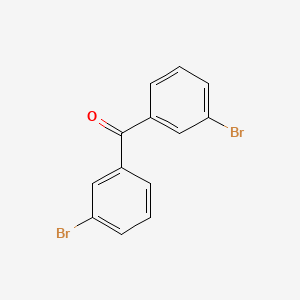
Bis(3-bromophenyl)methanone
Overview
Description
Bis(3-bromophenyl)methanone is an organic compound with the molecular formula C13H8Br2O . It is also known as 3,3’-Dibromobenzophenone . It is a ketone, which is a class of important organic synthesis intermediates .
Molecular Structure Analysis
The molecular structure of Bis(3-bromophenyl)methanone consists of 13 carbon atoms, 8 hydrogen atoms, 2 bromine atoms, and 1 oxygen atom . The average mass is 340.010 Da and the monoisotopic mass is 337.894165 Da .Physical And Chemical Properties Analysis
Bis(3-bromophenyl)methanone is a solid at 20 degrees Celsius . It appears as a white to light yellow powder or crystal . The melting point ranges from 144.0 to 148.0 degrees Celsius . The maximum absorption wavelength is 265 nm in CH2Cl2 .Scientific Research Applications
Material Science
This compound finds applications in material science, particularly in the development of organic frame materials. Its rigid and planar structure can contribute to the stability and rigidity of materials, which is essential in creating high-performance polymers and plastics .
Photophysical Studies
Bis(3-bromophenyl)methanone: can be used in photophysical studies due to its ability to absorb and emit light. Researchers can study its absorption and emission spectra to understand the solvent effects on these properties, which is crucial for designing compounds for optical applications .
Halogen Bonding Investigations
The presence of bromine atoms in Bis(3-bromophenyl)methanone makes it a suitable candidate for studying halogen bonding interactions. These interactions are a type of non-covalent interaction that is significant in crystal engineering and the design of supramolecular structures .
Mechanism of Action
Target of Action
Bis(3-bromophenyl)methanone is a type of symmetrical difenylketone It’s known that many natural products and active pharmaceutical ingredients contain symmetrical difenylketone structures .
Mode of Action
It’s known that this compound belongs to the class of organic compounds known as aryl-phenylketones . These are aromatic compounds containing a ketone substituted by one aryl group, and a phenyl group
Pharmacokinetics
Some pharmacokinetic properties can be inferred from its physicochemical properties . It has high gastrointestinal absorption and is BBB permeant, suggesting good bioavailability . It’s also an inhibitor of several cytochrome P450 enzymes, including CYP1A2, CYP2C19, and CYP2C9 , which could impact its metabolism and interactions with other drugs.
Result of Action
Given its physicochemical properties and the general activities of aryl-phenylketones , it’s likely to interact with various cellular targets, leading to a range of potential effects
properties
IUPAC Name |
bis(3-bromophenyl)methanone | |
---|---|---|
Details | Computed by Lexichem TK 2.7.0 (PubChem release 2021.05.07) | |
Source | PubChem | |
URL | https://pubchem.ncbi.nlm.nih.gov | |
Description | Data deposited in or computed by PubChem | |
InChI |
InChI=1S/C13H8Br2O/c14-11-5-1-3-9(7-11)13(16)10-4-2-6-12(15)8-10/h1-8H | |
Details | Computed by InChI 1.0.6 (PubChem release 2021.05.07) | |
Source | PubChem | |
URL | https://pubchem.ncbi.nlm.nih.gov | |
Description | Data deposited in or computed by PubChem | |
InChI Key |
QBNTVYGGZGPJDZ-UHFFFAOYSA-N | |
Details | Computed by InChI 1.0.6 (PubChem release 2021.05.07) | |
Source | PubChem | |
URL | https://pubchem.ncbi.nlm.nih.gov | |
Description | Data deposited in or computed by PubChem | |
Canonical SMILES |
C1=CC(=CC(=C1)Br)C(=O)C2=CC(=CC=C2)Br | |
Details | Computed by OEChem 2.3.0 (PubChem release 2021.05.07) | |
Source | PubChem | |
URL | https://pubchem.ncbi.nlm.nih.gov | |
Description | Data deposited in or computed by PubChem | |
Molecular Formula |
C13H8Br2O | |
Details | Computed by PubChem 2.1 (PubChem release 2021.05.07) | |
Source | PubChem | |
URL | https://pubchem.ncbi.nlm.nih.gov | |
Description | Data deposited in or computed by PubChem | |
Molecular Weight |
340.01 g/mol | |
Details | Computed by PubChem 2.1 (PubChem release 2021.05.07) | |
Source | PubChem | |
URL | https://pubchem.ncbi.nlm.nih.gov | |
Description | Data deposited in or computed by PubChem | |
Product Name |
Bis(3-bromophenyl)methanone |
Synthesis routes and methods I
Procedure details
Synthesis routes and methods II
Procedure details
Disclaimer and Information on In-Vitro Research Products
Please be aware that all articles and product information presented on BenchChem are intended solely for informational purposes. The products available for purchase on BenchChem are specifically designed for in-vitro studies, which are conducted outside of living organisms. In-vitro studies, derived from the Latin term "in glass," involve experiments performed in controlled laboratory settings using cells or tissues. It is important to note that these products are not categorized as medicines or drugs, and they have not received approval from the FDA for the prevention, treatment, or cure of any medical condition, ailment, or disease. We must emphasize that any form of bodily introduction of these products into humans or animals is strictly prohibited by law. It is essential to adhere to these guidelines to ensure compliance with legal and ethical standards in research and experimentation.