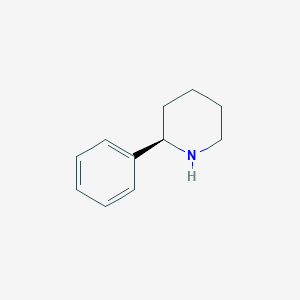
(R)-2-phenylpiperidine
Overview
Description
(R)-2-Phenylpiperidine is a cyclic organic compound belonging to the class of piperidine compounds. It is an important chemical intermediate used in the synthesis of many compounds and drugs, including analgesics, anti-depressants, and anti-psychotics. It is also used in the synthesis of a variety of other compounds, such as dyes, fragrances, and food additives. In addition, (R)-2-phenylpiperidine has recently been studied for its potential therapeutic applications in the treatment of various diseases, including cancer, diabetes, and Alzheimer’s disease.
Scientific Research Applications
Dopamine Receptor Research
- (R)-2-phenylpiperidine derivatives, specifically OSU6162 and ACR16, have been investigated for their dopamine stabilizing effects and potential use in treating brain disorders such as schizophrenia and Huntington's disease. These studies focus on the in vitro activities of these compounds at the D2 dopamine receptor (Kara, Lin, Svensson, Johansson, & Strange, 2010).
Antibacterial Research
- Research has been conducted on the effects of isomeric non-antibiotic depressants (DPR), phenothiazines, and thixenes, as well as antidepressant (ADPR) phenylpiperidine neurotropic drugs, in combination with classical antimicrobials. These compounds, including isomers of phenylpiperidines, have shown promise in combatting multidrug-resistant microorganisms like Staphylococcus aureus and Streptococcus pneumoniae (Kristiansen, Hendricks, Delvin, Butterworth, Aagaard, Christensen, Flores, & Keyzer, 2007).
NMDA Receptor Antagonists Development
- Phenylpiperidine compounds, based on benzylpiperidine and phenylpiperidine templates, have been developed as NR2B antagonists, showing efficacy in neuroprotection, anti-hyperalgesic, and anti-Parkinson animal models. These findings are crucial for therapeutic applications in various pathophysiological conditions (Nikam & Meltzer, 2002).
Antidepressant Efficacy Studies
- Phenylpiperidine derivatives have been evaluated for their antidepressant properties. For example, racemic phenibut and its optical isomers have been tested in pharmacological assessments, revealing that R-phenibut is more potent than racemic phenibut in most tests, including the forced swimming test and tail-flick test for analgesic activity (Dambrova, Zvejniece, Liepinsh, Cirule, Zharkova, Veinberg, & Kalvinsh, 2008).
Chemical Synthesis and Alkaloid Synthesis
- δ-Amino β-keto esters have been designed as polyfunctionalized chiral building blocks for alkaloid synthesis, including the asymmetric synthesis of (R)-(+)-2-Phenylpiperidine. This showcases the utility of these compounds in the field of synthetic chemistry and pharmaceuticals (Davis, Chao, Fang, & Szewczyk, 2000).
properties
IUPAC Name |
(2R)-2-phenylpiperidine | |
---|---|---|
Details | Computed by LexiChem 2.6.6 (PubChem release 2019.06.18) | |
Source | PubChem | |
URL | https://pubchem.ncbi.nlm.nih.gov | |
Description | Data deposited in or computed by PubChem | |
InChI |
InChI=1S/C11H15N/c1-2-6-10(7-3-1)11-8-4-5-9-12-11/h1-3,6-7,11-12H,4-5,8-9H2/t11-/m1/s1 | |
Details | Computed by InChI 1.0.5 (PubChem release 2019.06.18) | |
Source | PubChem | |
URL | https://pubchem.ncbi.nlm.nih.gov | |
Description | Data deposited in or computed by PubChem | |
InChI Key |
WGIAUTGOUJDVEI-LLVKDONJSA-N | |
Details | Computed by InChI 1.0.5 (PubChem release 2019.06.18) | |
Source | PubChem | |
URL | https://pubchem.ncbi.nlm.nih.gov | |
Description | Data deposited in or computed by PubChem | |
Canonical SMILES |
C1CCNC(C1)C2=CC=CC=C2 | |
Details | Computed by OEChem 2.1.5 (PubChem release 2019.06.18) | |
Source | PubChem | |
URL | https://pubchem.ncbi.nlm.nih.gov | |
Description | Data deposited in or computed by PubChem | |
Isomeric SMILES |
C1CCN[C@H](C1)C2=CC=CC=C2 | |
Details | Computed by OEChem 2.1.5 (PubChem release 2019.06.18) | |
Source | PubChem | |
URL | https://pubchem.ncbi.nlm.nih.gov | |
Description | Data deposited in or computed by PubChem | |
Molecular Formula |
C11H15N | |
Details | Computed by PubChem 2.1 (PubChem release 2019.06.18) | |
Source | PubChem | |
URL | https://pubchem.ncbi.nlm.nih.gov | |
Description | Data deposited in or computed by PubChem | |
Molecular Weight |
161.24 g/mol | |
Details | Computed by PubChem 2.1 (PubChem release 2021.05.07) | |
Source | PubChem | |
URL | https://pubchem.ncbi.nlm.nih.gov | |
Description | Data deposited in or computed by PubChem | |
Product Name |
(R)-2-phenylpiperidine |
Synthesis routes and methods
Procedure details
Disclaimer and Information on In-Vitro Research Products
Please be aware that all articles and product information presented on BenchChem are intended solely for informational purposes. The products available for purchase on BenchChem are specifically designed for in-vitro studies, which are conducted outside of living organisms. In-vitro studies, derived from the Latin term "in glass," involve experiments performed in controlled laboratory settings using cells or tissues. It is important to note that these products are not categorized as medicines or drugs, and they have not received approval from the FDA for the prevention, treatment, or cure of any medical condition, ailment, or disease. We must emphasize that any form of bodily introduction of these products into humans or animals is strictly prohibited by law. It is essential to adhere to these guidelines to ensure compliance with legal and ethical standards in research and experimentation.