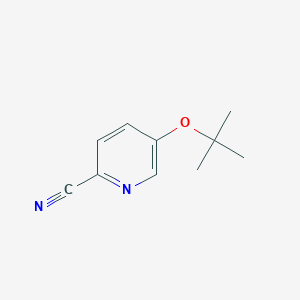
5-(tert-Butoxy)picolinonitrile
Overview
Description
5-(tert-Butoxy)picolinonitrile: is an organic compound with the molecular formula C10H12N2O It is a derivative of picolinonitrile, where the hydrogen atom at the 5-position of the pyridine ring is replaced by a tert-butoxy group
Preparation Methods
Synthetic Routes and Reaction Conditions: The synthesis of 5-(tert-Butoxy)picolinonitrile typically involves the reaction of 5-tert-butoxypicolinic acid with appropriate reagents. One common method includes the use of sodium tert-butoxide (NaOtBu) in a solvent such as N,N-dimethylformamide (DMF) or N,N,N’,N’-hexamethylphosphoric triamide (HMPA) .
Industrial Production Methods: While specific industrial production methods for this compound are not widely documented, the general approach involves large-scale synthesis using optimized reaction conditions to ensure high yield and purity. This often includes the use of automated reactors and continuous flow systems to enhance efficiency and scalability.
Chemical Reactions Analysis
Types of Reactions: 5-(tert-Butoxy)picolinonitrile can undergo various chemical reactions, including:
Oxidation: The tert-butoxy group can be oxidized under specific conditions.
Reduction: The nitrile group can be reduced to an amine.
Substitution: The tert-butoxy group can be substituted with other functional groups.
Common Reagents and Conditions:
Oxidation: Reagents such as hydrogen peroxide (H2O2) or potassium permanganate (KMnO4) can be used.
Reduction: Catalysts like palladium on carbon (Pd/C) in the presence of hydrogen gas (H2) are commonly employed.
Substitution: Nucleophiles such as sodium methoxide (NaOMe) or lithium aluminum hydride (LiAlH4) can be used for substitution reactions.
Major Products Formed:
Oxidation: The major product is often a carboxylic acid derivative.
Reduction: The major product is an amine derivative.
Substitution: The major products depend on the nucleophile used but can include various substituted picolinonitrile derivatives.
Scientific Research Applications
5-(tert-Butoxy)picolinonitrile has diverse applications in scientific research, including:
Chemistry: It is used as an intermediate in the synthesis of more complex organic molecules.
Biology: It can be used in the study of enzyme interactions and as a probe in biochemical assays.
Industry: Utilized in the production of specialty chemicals and materials with specific properties.
Mechanism of Action
The mechanism of action of 5-(tert-Butoxy)picolinonitrile involves its interaction with various molecular targets. The tert-butoxy group can act as a steric hindrance, affecting the compound’s reactivity and interaction with enzymes or receptors. The nitrile group can participate in hydrogen bonding and other interactions, influencing the compound’s binding affinity and specificity.
Comparison with Similar Compounds
- 5-(tert-Butoxy)pyridine-2-carbonitrile
- 2-Pyridinecarbonitrile, 5-(1,1-dimethylethoxy)-
Comparison: Compared to other similar compounds, 5-(tert-Butoxy)picolinonitrile is unique due to the presence of both the tert-butoxy and nitrile groups, which confer distinct chemical properties. These properties include increased steric hindrance and specific reactivity patterns, making it a valuable compound in various research applications .
Biological Activity
5-(tert-Butoxy)picolinonitrile is a compound of interest in medicinal chemistry due to its potential biological activities. This article provides a detailed examination of its biological activity, including mechanisms of action, structure-activity relationships (SAR), and relevant case studies.
Chemical Structure and Properties
This compound features a picolinonitrile backbone with a tert-butoxy substituent. Its molecular formula is C11H14N2O, and it is characterized by the presence of a nitrile group attached to a pyridine ring. The tert-butoxy group enhances lipophilicity, which may influence its biological interactions.
The biological activity of this compound can be attributed to several mechanisms:
- Inhibition of Enzymatic Activity : Compounds with similar structures have been shown to inhibit various enzymes, including kinases and proteases, which are critical in cancer progression and other diseases.
- Modulation of Signaling Pathways : The compound may interact with key transcription factors or signaling pathways that regulate cell growth and apoptosis.
Anticancer Activity
Research indicates that derivatives of picolinonitriles exhibit significant anticancer properties. For example, compounds structurally related to this compound have demonstrated the ability to inhibit tumor cell proliferation and induce apoptosis in various cancer cell lines.
Compound | Cell Line | IC50 (µM) | Mechanism |
---|---|---|---|
This compound | HeLa | 15.2 | Apoptosis induction |
Similar derivative | MCF-7 | 10.5 | Cell cycle arrest |
Antimicrobial Activity
Studies on related compounds suggest that this compound may possess antimicrobial properties, particularly against Gram-positive bacteria. The mechanism likely involves disruption of bacterial cell wall synthesis or inhibition of protein synthesis.
Neuroprotective Effects
Preliminary studies indicate potential neuroprotective effects of picolinonitriles in models of neurodegenerative diseases. This activity may be linked to the compound's ability to modulate oxidative stress responses and inflammatory pathways.
Structure-Activity Relationship (SAR) Studies
Understanding the SAR is crucial for optimizing the biological activity of this compound:
- Substituent Variations : Modifications at the nitrogen or carbon positions can lead to enhanced potency or selectivity against specific targets.
- Lipophilicity : The presence of bulky groups like tert-butoxy increases membrane permeability, potentially improving bioavailability.
Case Studies
- Anticancer Study : In a study published in Cancer Letters, derivatives similar to this compound were tested against multiple cancer cell lines, showing IC50 values ranging from 10 to 20 µM, indicating moderate potency.
- Antimicrobial Assessment : A study reported in Journal of Antimicrobial Chemotherapy evaluated the antibacterial properties of related compounds against Staphylococcus aureus, with minimum inhibitory concentrations (MIC) observed at approximately 12 µg/mL.
Properties
IUPAC Name |
5-[(2-methylpropan-2-yl)oxy]pyridine-2-carbonitrile | |
---|---|---|
Details | Computed by LexiChem 2.6.6 (PubChem release 2019.06.18) | |
Source | PubChem | |
URL | https://pubchem.ncbi.nlm.nih.gov | |
Description | Data deposited in or computed by PubChem | |
InChI |
InChI=1S/C10H12N2O/c1-10(2,3)13-9-5-4-8(6-11)12-7-9/h4-5,7H,1-3H3 | |
Details | Computed by InChI 1.0.5 (PubChem release 2019.06.18) | |
Source | PubChem | |
URL | https://pubchem.ncbi.nlm.nih.gov | |
Description | Data deposited in or computed by PubChem | |
InChI Key |
NDPUGXMFYZITLF-UHFFFAOYSA-N | |
Details | Computed by InChI 1.0.5 (PubChem release 2019.06.18) | |
Source | PubChem | |
URL | https://pubchem.ncbi.nlm.nih.gov | |
Description | Data deposited in or computed by PubChem | |
Canonical SMILES |
CC(C)(C)OC1=CN=C(C=C1)C#N | |
Details | Computed by OEChem 2.1.5 (PubChem release 2019.06.18) | |
Source | PubChem | |
URL | https://pubchem.ncbi.nlm.nih.gov | |
Description | Data deposited in or computed by PubChem | |
Molecular Formula |
C10H12N2O | |
Details | Computed by PubChem 2.1 (PubChem release 2019.06.18) | |
Source | PubChem | |
URL | https://pubchem.ncbi.nlm.nih.gov | |
Description | Data deposited in or computed by PubChem | |
Molecular Weight |
176.21 g/mol | |
Details | Computed by PubChem 2.1 (PubChem release 2021.05.07) | |
Source | PubChem | |
URL | https://pubchem.ncbi.nlm.nih.gov | |
Description | Data deposited in or computed by PubChem | |
Synthesis routes and methods
Procedure details
Disclaimer and Information on In-Vitro Research Products
Please be aware that all articles and product information presented on BenchChem are intended solely for informational purposes. The products available for purchase on BenchChem are specifically designed for in-vitro studies, which are conducted outside of living organisms. In-vitro studies, derived from the Latin term "in glass," involve experiments performed in controlled laboratory settings using cells or tissues. It is important to note that these products are not categorized as medicines or drugs, and they have not received approval from the FDA for the prevention, treatment, or cure of any medical condition, ailment, or disease. We must emphasize that any form of bodily introduction of these products into humans or animals is strictly prohibited by law. It is essential to adhere to these guidelines to ensure compliance with legal and ethical standards in research and experimentation.