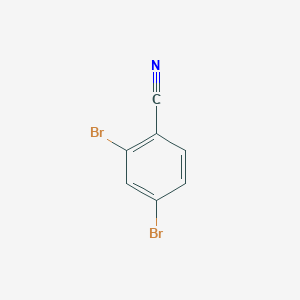
2,4-Dibromobenzonitrile
Overview
Description
2,4-Dibromobenzonitrile is an organic compound with the molecular formula C7H3Br2N. It is a derivative of benzonitrile, where two bromine atoms are substituted at the 2nd and 4th positions of the benzene ring. This compound is known for its applications in organic synthesis and as an intermediate in the production of various chemicals.
Scientific Research Applications
2,4-Dibromobenzonitrile is widely used in scientific research due to its versatility:
Chemistry: It serves as a building block in the synthesis of complex organic molecules and pharmaceuticals.
Biology: It is used in the study of enzyme inhibitors and as a precursor for bioactive compounds.
Medicine: It is investigated for its potential therapeutic properties and as an intermediate in drug synthesis.
Industry: It is used in the production of dyes, agrochemicals, and specialty chemicals.
Safety and Hazards
2,4-Dibromobenzonitrile is classified as a warning hazard according to the Globally Harmonized System of Classification and Labelling of Chemicals (GHS) . It is harmful if swallowed or in contact with skin . Protective measures such as wearing protective gloves, clothing, eye protection, and face protection are recommended .
Preparation Methods
Synthetic Routes and Reaction Conditions: 2,4-Dibromobenzonitrile can be synthesized through several methods. One common approach involves the bromination of benzonitrile. The reaction typically uses bromine (Br2) in the presence of a catalyst such as iron (Fe) or aluminum bromide (AlBr3). The reaction is carried out under controlled conditions to ensure selective bromination at the 2nd and 4th positions.
Industrial Production Methods: In industrial settings, the production of this compound often involves large-scale bromination processes. The reaction conditions are optimized to achieve high yields and purity. The use of continuous flow reactors and advanced separation techniques helps in the efficient production of this compound.
Chemical Reactions Analysis
Types of Reactions: 2,4-Dibromobenzonitrile undergoes various chemical reactions, including:
Substitution Reactions: It can participate in nucleophilic substitution reactions where the bromine atoms are replaced by other nucleophiles.
Reduction Reactions: The nitrile group can be reduced to an amine using reducing agents such as lithium aluminum hydride (LiAlH4).
Coupling Reactions: It can undergo coupling reactions with arylboronic acids in the presence of palladium catalysts to form biaryl compounds.
Common Reagents and Conditions:
Nucleophilic Substitution: Sodium hydroxide (NaOH) or potassium hydroxide (KOH) in an aqueous or alcoholic medium.
Reduction: Lithium aluminum hydride (LiAlH4) in anhydrous ether.
Coupling: Palladium catalysts such as tetrakis(triphenylphosphine)palladium(0) in toluene or ethanol.
Major Products:
Substitution: Formation of substituted benzonitriles.
Reduction: Formation of 2,4-dibromoaniline.
Coupling: Formation of biaryl compounds.
Mechanism of Action
The mechanism of action of 2,4-Dibromobenzonitrile depends on its application. In organic synthesis, it acts as an electrophile in substitution reactions. In biological systems, it may interact with specific enzymes or receptors, leading to inhibition or activation of biological pathways. The exact molecular targets and pathways vary based on the specific application and context of use.
Comparison with Similar Compounds
2,4-Dichlorobenzonitrile: Similar structure with chlorine atoms instead of bromine.
3,4-Dibromobenzonitrile: Bromine atoms substituted at the 3rd and 4th positions.
2,6-Dibromobenzonitrile: Bromine atoms substituted at the 2nd and 6th positions.
Uniqueness: 2,4-Dibromobenzonitrile is unique due to its specific substitution pattern, which imparts distinct reactivity and properties. The presence of bromine atoms at the 2nd and 4th positions makes it a valuable intermediate in organic synthesis, offering different reactivity compared to its chlorinated or differently substituted counterparts.
Properties
IUPAC Name |
2,4-dibromobenzonitrile | |
---|---|---|
Details | Computed by LexiChem 2.6.6 (PubChem release 2019.06.18) | |
Source | PubChem | |
URL | https://pubchem.ncbi.nlm.nih.gov | |
Description | Data deposited in or computed by PubChem | |
InChI |
InChI=1S/C7H3Br2N/c8-6-2-1-5(4-10)7(9)3-6/h1-3H | |
Details | Computed by InChI 1.0.5 (PubChem release 2019.06.18) | |
Source | PubChem | |
URL | https://pubchem.ncbi.nlm.nih.gov | |
Description | Data deposited in or computed by PubChem | |
InChI Key |
MIQRQLRZDQTHGM-UHFFFAOYSA-N | |
Details | Computed by InChI 1.0.5 (PubChem release 2019.06.18) | |
Source | PubChem | |
URL | https://pubchem.ncbi.nlm.nih.gov | |
Description | Data deposited in or computed by PubChem | |
Canonical SMILES |
C1=CC(=C(C=C1Br)Br)C#N | |
Details | Computed by OEChem 2.1.5 (PubChem release 2019.06.18) | |
Source | PubChem | |
URL | https://pubchem.ncbi.nlm.nih.gov | |
Description | Data deposited in or computed by PubChem | |
Molecular Formula |
C7H3Br2N | |
Details | Computed by PubChem 2.1 (PubChem release 2019.06.18) | |
Source | PubChem | |
URL | https://pubchem.ncbi.nlm.nih.gov | |
Description | Data deposited in or computed by PubChem | |
Molecular Weight |
260.91 g/mol | |
Details | Computed by PubChem 2.1 (PubChem release 2021.05.07) | |
Source | PubChem | |
URL | https://pubchem.ncbi.nlm.nih.gov | |
Description | Data deposited in or computed by PubChem | |
Synthesis routes and methods I
Procedure details
Synthesis routes and methods II
Procedure details
Synthesis routes and methods III
Procedure details
Disclaimer and Information on In-Vitro Research Products
Please be aware that all articles and product information presented on BenchChem are intended solely for informational purposes. The products available for purchase on BenchChem are specifically designed for in-vitro studies, which are conducted outside of living organisms. In-vitro studies, derived from the Latin term "in glass," involve experiments performed in controlled laboratory settings using cells or tissues. It is important to note that these products are not categorized as medicines or drugs, and they have not received approval from the FDA for the prevention, treatment, or cure of any medical condition, ailment, or disease. We must emphasize that any form of bodily introduction of these products into humans or animals is strictly prohibited by law. It is essential to adhere to these guidelines to ensure compliance with legal and ethical standards in research and experimentation.