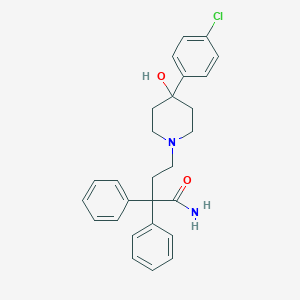
N-Didesmethyl Loperamide
Overview
Description
N-didesmethyl Loperamide: is a metabolite of loperamide, a well-known anti-diarrheal medication. Loperamide is a synthetic opioid receptor agonist that primarily acts on the μ-opioid receptors in the gastrointestinal tract to reduce motility and increase absorption of fluids and electrolytes . This compound is formed through the metabolic process of loperamide and retains some of its pharmacological properties .
Mechanism of Action
Target of Action
N-Didesmethyl Loperamide is an active metabolite of the peripheral μ1-opioid receptor agonist loperamide . The μ1-opioid receptor is primarily found in the brain and is known to play a crucial role in pain relief, respiratory depression, and euphoria .
Mode of Action
This compound acts as an agonist at the μ1-opioid receptor . It binds to these receptors and activates them, leading to a series of downstream effects. Specifically, it impedes electrically induced contractions in isolated guinea pig myenteric plexus .
Biochemical Pathways
The activation of the μ1-opioid receptor by this compound leads to a decrease in the release of neurotransmitters such as substance P, which is involved in the transmission of pain signals . This results in a decrease in the perception of pain.
Pharmacokinetics
This compound is metabolized primarily through oxidative N-demethylation mediated by CYP2C8 and CYP3A4, forming N-demethyl loperamide . CYP2B6 and CYP2D6 also play a minor role in its metabolism . The metabolites of loperamide, including this compound, are pharmacologically inactive .
Result of Action
The primary result of this compound’s action is the inhibition of electrically induced contractions in isolated guinea pig myenteric plexus . Additionally, it enhances the sensitivity of a chloroquine-resistant P. falciparum strain to chloroquine .
Action Environment
The action of this compound can be influenced by various environmental factors. For instance, the presence of other drugs that inhibit or induce CYP2C8, CYP3A4, CYP2B6, or CYP2D6 could potentially affect the metabolism and efficacy of this compound
Biochemical Analysis
Biochemical Properties
N-Didesmethyl Loperamide interacts with several enzymes and proteins. It inhibits electricity-induced contractions in isolated guinea pig myenteric plexus . It also sensitizes a chloroquine-resistant P. falciparum strain to chloroquine .
Cellular Effects
This compound has been shown to have effects on various types of cells. For instance, it has been found to induce changes in glioblastoma cells . It also has an impact on cell signaling pathways, gene expression, and cellular metabolism .
Molecular Mechanism
This compound exerts its effects at the molecular level through various mechanisms. It is known to bind with high affinity and selectivity to the μ opioid receptor . It also has a role in enzyme inhibition or activation, and changes in gene expression .
Temporal Effects in Laboratory Settings
The effects of this compound change over time in laboratory settings. For instance, it has been found that high urine concentrations of this compound can produce positive immunoassay drug screen results for 2 urine fentanyl assays, and 1 urine buprenorphine assay .
Dosage Effects in Animal Models
The effects of this compound vary with different dosages in animal models. For example, it has been shown to induce psychosis in rodents and humans at certain concentrations .
Metabolic Pathways
This compound is involved in several metabolic pathways. It is metabolized primarily through oxidative N-demethylation mediated by CYP2C8 and CYP3A4, to form N-demethyl loperamide .
Preparation Methods
Synthetic Routes and Reaction Conditions: : The synthesis of N-didesmethyl Loperamide involves the demethylation of loperamide. This process can be achieved through various chemical reactions, including the use of strong acids or bases to remove the methyl groups from the nitrogen atoms in the loperamide molecule .
Industrial Production Methods: : Industrial production of this compound typically involves large-scale chemical synthesis using optimized reaction conditions to ensure high yield and purity. The process may include steps such as purification through chromatography and crystallization to isolate the desired compound .
Chemical Reactions Analysis
Types of Reactions: : N-didesmethyl Loperamide can undergo several types of chemical reactions, including:
Oxidation: This reaction involves the addition of oxygen or the removal of hydrogen from the compound.
Reduction: This reaction involves the addition of hydrogen or the removal of oxygen from the compound.
Substitution: This reaction involves the replacement of one functional group with another.
Common Reagents and Conditions
Oxidation: Common reagents include potassium permanganate (KMnO4) and hydrogen peroxide (H2O2).
Reduction: Common reagents include lithium aluminum hydride (LiAlH4) and sodium borohydride (NaBH4).
Substitution: Common reagents include halogens (e.g., chlorine, bromine) and nucleophiles (e.g., hydroxide, cyanide).
Major Products: : The major products formed from these reactions depend on the specific reagents and conditions used. For example, oxidation may produce this compound oxide, while reduction may yield a more saturated derivative.
Scientific Research Applications
Chemistry: : N-didesmethyl Loperamide is used as a reference standard in analytical chemistry for the quantification of loperamide and its metabolites in biological samples .
Biology: : In biological research, this compound is studied for its interactions with opioid receptors and its potential effects on gastrointestinal motility .
Medicine: : this compound is investigated for its potential therapeutic applications, including its use as an anti-diarrheal agent and its effects on opioid receptors .
Industry: : In the pharmaceutical industry, this compound is used in the development and testing of new drugs that target opioid receptors .
Comparison with Similar Compounds
Similar Compounds
Loperamide: The parent compound of N-didesmethyl Loperamide, used as an anti-diarrheal medication.
N-desmethyl Loperamide: Another metabolite of loperamide with similar pharmacological properties.
Uniqueness: : N-didesmethyl Loperamide is unique in its specific binding affinity and selectivity for μ-opioid receptors, which makes it a valuable compound for studying opioid receptor interactions and developing new therapeutic agents .
Biological Activity
N-Didesmethyl Loperamide, a metabolite of the widely used anti-diarrheal agent loperamide, has garnered attention due to its pharmacological properties and potential implications in toxicity. This article explores the biological activity of this compound, focusing on its mechanisms of action, metabolic pathways, case studies illustrating its clinical impact, and comparative analyses with its parent compound, loperamide.
This compound is primarily formed through the N-demethylation of loperamide, which is mediated by cytochrome P450 enzymes, particularly CYP2C8 and CYP3A4 . While loperamide acts as a μ-opioid receptor agonist in the gastrointestinal tract to reduce motility and increase water absorption, this compound exhibits a significantly lower affinity for these receptors. Research indicates that it is a weaker inhibitor of the hERG potassium channel compared to loperamide, suggesting a reduced risk of cardiotoxicity .
Pharmacological Profile
- Receptor Binding : this compound shows less than 50% inhibition at various receptors at a concentration of 10 μM, indicating lower potency compared to loperamide .
- Cardiac Ion Channels : It has been shown to inhibit cardiac ion channels such as hERG but at much lower levels than loperamide (7.5-fold weaker) which may contribute to its reduced cardiotoxic profile .
Metabolism and Pharmacokinetics
The metabolism of loperamide leads to several metabolites, with this compound being one of the most significant. The pharmacokinetics of loperamide indicate that it undergoes extensive first-pass metabolism, resulting in low systemic bioavailability (approximately 0.3%) and minimal central nervous system effects under normal therapeutic doses . However, in cases of overdose or misuse, such as in chronic diarrhea treatment or opioid withdrawal management, elevated levels of both loperamide and its metabolites can lead to severe adverse effects.
Case Study 1: Loperamide-Induced Cardiogenic Syncope
A notable case involved a 25-year-old female who experienced multiple syncopal episodes attributed to excessive loperamide use (100–150 tablets daily). Electrocardiograms revealed a Brugada-like pattern and subsequent cardiogenic shock. After identifying her high loperamide levels (190 ng/mL) and its metabolite (520 ng/mL), she was treated with intralipid emulsion therapy and supportive care, ultimately recovering from her cardiac complications .
Timeline | Event |
---|---|
Day 1 | Syncopal episodes; ECG shows VT |
Day 7 | Cardiogenic shock; elevated loperamide levels identified |
Day 10 | Recovery after treatment; loperamide undetectable |
Case Study 2: Ventricular Storm Induced by Loperamide
Another case reported a 29-year-old female who ingested approximately 600 mg of loperamide daily for chronic gastrointestinal issues. She presented with tachycardia and prolonged QTc interval leading to torsades de pointes. Treatment involved aggressive management in the cardiac intensive care unit, highlighting the risks associated with high doses of loperamide and its metabolites .
Comparative Analysis: this compound vs. Loperamide
The following table summarizes key differences between this compound and its parent compound:
Parameter | This compound | Loperamide |
---|---|---|
Receptor Affinity | Weaker μ-opioid agonist | Strong μ-opioid agonist |
hERG Inhibition | 7.5-fold weaker | High affinity |
Cardiotoxicity Risk | Lower | Higher |
Bioavailability | Not extensively studied | ~0.3% |
Metabolic Pathway | Formed via N-demethylation | Extensive first-pass metabolism |
Properties
IUPAC Name |
4-[4-(4-chlorophenyl)-4-hydroxypiperidin-1-yl]-2,2-diphenylbutanamide | |
---|---|---|
Source | PubChem | |
URL | https://pubchem.ncbi.nlm.nih.gov | |
Description | Data deposited in or computed by PubChem | |
InChI |
InChI=1S/C27H29ClN2O2/c28-24-13-11-21(12-14-24)26(32)15-18-30(19-16-26)20-17-27(25(29)31,22-7-3-1-4-8-22)23-9-5-2-6-10-23/h1-14,32H,15-20H2,(H2,29,31) | |
Source | PubChem | |
URL | https://pubchem.ncbi.nlm.nih.gov | |
Description | Data deposited in or computed by PubChem | |
InChI Key |
PXJHDOGGBLQFRX-UHFFFAOYSA-N | |
Source | PubChem | |
URL | https://pubchem.ncbi.nlm.nih.gov | |
Description | Data deposited in or computed by PubChem | |
Canonical SMILES |
C1CN(CCC1(C2=CC=C(C=C2)Cl)O)CCC(C3=CC=CC=C3)(C4=CC=CC=C4)C(=O)N | |
Source | PubChem | |
URL | https://pubchem.ncbi.nlm.nih.gov | |
Description | Data deposited in or computed by PubChem | |
Molecular Formula |
C27H29ClN2O2 | |
Source | PubChem | |
URL | https://pubchem.ncbi.nlm.nih.gov | |
Description | Data deposited in or computed by PubChem | |
DSSTOX Substance ID |
DTXSID10648790 | |
Record name | 4-[4-(4-Chlorophenyl)-4-hydroxypiperidin-1-yl]-2,2-diphenylbutanamide | |
Source | EPA DSSTox | |
URL | https://comptox.epa.gov/dashboard/DTXSID10648790 | |
Description | DSSTox provides a high quality public chemistry resource for supporting improved predictive toxicology. | |
Molecular Weight |
449.0 g/mol | |
Source | PubChem | |
URL | https://pubchem.ncbi.nlm.nih.gov | |
Description | Data deposited in or computed by PubChem | |
CAS No. |
66164-06-5 | |
Record name | 4-[4-(4-Chlorophenyl)-4-hydroxypiperidin-1-yl]-2,2-diphenylbutanamide | |
Source | EPA DSSTox | |
URL | https://comptox.epa.gov/dashboard/DTXSID10648790 | |
Description | DSSTox provides a high quality public chemistry resource for supporting improved predictive toxicology. | |
Synthesis routes and methods
Procedure details
Retrosynthesis Analysis
AI-Powered Synthesis Planning: Our tool employs the Template_relevance Pistachio, Template_relevance Bkms_metabolic, Template_relevance Pistachio_ringbreaker, Template_relevance Reaxys, Template_relevance Reaxys_biocatalysis model, leveraging a vast database of chemical reactions to predict feasible synthetic routes.
One-Step Synthesis Focus: Specifically designed for one-step synthesis, it provides concise and direct routes for your target compounds, streamlining the synthesis process.
Accurate Predictions: Utilizing the extensive PISTACHIO, BKMS_METABOLIC, PISTACHIO_RINGBREAKER, REAXYS, REAXYS_BIOCATALYSIS database, our tool offers high-accuracy predictions, reflecting the latest in chemical research and data.
Strategy Settings
Precursor scoring | Relevance Heuristic |
---|---|
Min. plausibility | 0.01 |
Model | Template_relevance |
Template Set | Pistachio/Bkms_metabolic/Pistachio_ringbreaker/Reaxys/Reaxys_biocatalysis |
Top-N result to add to graph | 6 |
Feasible Synthetic Routes
Disclaimer and Information on In-Vitro Research Products
Please be aware that all articles and product information presented on BenchChem are intended solely for informational purposes. The products available for purchase on BenchChem are specifically designed for in-vitro studies, which are conducted outside of living organisms. In-vitro studies, derived from the Latin term "in glass," involve experiments performed in controlled laboratory settings using cells or tissues. It is important to note that these products are not categorized as medicines or drugs, and they have not received approval from the FDA for the prevention, treatment, or cure of any medical condition, ailment, or disease. We must emphasize that any form of bodily introduction of these products into humans or animals is strictly prohibited by law. It is essential to adhere to these guidelines to ensure compliance with legal and ethical standards in research and experimentation.