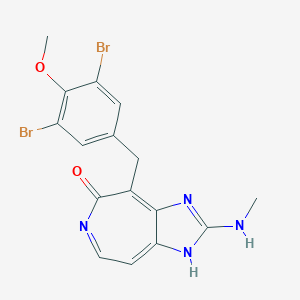
Ceratamine B
Overview
Description
Ceratamine B is a naturally occurring compound that has been used in scientific research for many years. It is a member of the ceramide family, which is composed of a variety of long-chain fatty acids. This compound is a small molecule that is highly soluble in water and has been used for a variety of research applications, including cell culture and drug delivery.
Scientific Research Applications
Antimitotic and Microtubule Disruption Properties : Ceratamines A and B disrupt microtubule dynamics, making them valuable in cancer drug discovery due to their antimitotic properties. They are particularly noted for their use in the therapeutic armamentarium, enriched by molecular biology and genomic sciences, providing new treatment options (Smith et al., 2014); (Drews, 2000).
Synthetic Analogs and Cellular Effects : As a synthetic antimitotic analog of ceratamine A, Ceratamine B exhibits unique cellular effects. In breast carcinoma MCF-7 cells, it causes a concentration-dependent block of cell cycle progression exclusively at mitosis (Nodwell et al., 2008); (Karjala et al., 2005).
Isolation from Marine Sponge and Antimitotic Effects : Ceratamines A and B, isolated from the marine sponge Pseudoceratina sp., are recognized as novel antimitotic heterocyclic alkaloids (Manzo et al., 2003).
Synthetic Routes and Tubulin-Binding : this compound can be synthesized using efficient synthetic routes, being a tubulin-binding agent. This aspect contributes to its potential as a therapeutic agent (Coleman et al., 2009).
Mechanism of Action
Target of Action
Ceratamine B is a heterocyclic alkaloid that primarily targets microtubules . Microtubules are a component of the cell’s cytoskeleton and play a crucial role in maintaining cell shape, enabling cell motility, and most importantly, in cell division .
Mode of Action
This compound interacts with its target by directly stimulating microtubule polymerization . This interaction results in a dense perinuclear microtubule network in interphase cells and multiple pillar-like tubulin structures in mitotic cells .
Biochemical Pathways
The primary biochemical pathway affected by this compound is the microtubule dynamics . By stabilizing microtubules, this compound disrupts their normal dynamics, leading to a block in cell cycle progression, specifically at mitosis . This disruption can ultimately lead to cell death, especially in highly proliferative cells like cancer cells .
Pharmacokinetics
These studies involved incubating the compound within rat and human liver microsomes . The initial analysis was performed in a qualitative manner with LC-MS/MS techniques used for structure elucidation .
Result of Action
The molecular and cellular effects of this compound’s action are primarily observed as changes in cell cycle progression. Treatment of breast carcinoma MCF-7 cells with this compound causes a concentration-dependent block of cell cycle progression exclusively at mitosis . This block in cell cycle progression can lead to cell death, particularly in cancer cells .
Action Environment
Biochemical Analysis
Biochemical Properties
Ceratamine B plays a crucial role in biochemical reactions by interacting with microtubules. It stabilizes microtubules, preventing their depolymerization, which is essential for cell division. This stabilization mechanism is similar to that of other well-known microtubule-targeting agents like taxanes. This compound interacts with tubulin, the protein subunit of microtubules, and enhances its polymerization. This interaction disrupts the normal dynamics of microtubules, leading to cell cycle arrest at the mitotic phase .
Cellular Effects
This compound exerts significant effects on various types of cells, particularly cancer cells. It induces cell cycle arrest at the G2/M phase, leading to apoptosis or programmed cell death. This compound affects cell signaling pathways, including the mitogen-activated protein kinase (MAPK) pathway, which is crucial for cell proliferation and survival. This compound also influences gene expression by upregulating pro-apoptotic genes and downregulating anti-apoptotic genes. Additionally, it impacts cellular metabolism by altering the balance between anabolic and catabolic processes .
Molecular Mechanism
The molecular mechanism of this compound involves its binding to the colchicine-binding site on tubulin. This binding inhibits the depolymerization of microtubules, leading to their stabilization. This compound also affects the activity of various enzymes involved in cell cycle regulation, such as cyclin-dependent kinases (CDKs). By inhibiting CDKs, this compound prevents the progression of the cell cycle, leading to cell cycle arrest and apoptosis. Furthermore, this compound modulates gene expression by interacting with transcription factors and other regulatory proteins .
Temporal Effects in Laboratory Settings
In laboratory settings, the effects of this compound change over time. Initially, it induces rapid cell cycle arrest and apoptosis in cancer cells. Prolonged exposure to this compound can lead to the development of resistance in some cell lines. The stability of this compound is also a critical factor, as it can degrade over time, reducing its efficacy. Long-term studies have shown that this compound can have lasting effects on cellular function, including alterations in cell morphology and gene expression patterns .
Dosage Effects in Animal Models
The effects of this compound vary with different dosages in animal models. At low doses, this compound effectively induces cell cycle arrest and apoptosis without causing significant toxicity. At higher doses, this compound can cause adverse effects, including neurotoxicity and hepatotoxicity. Threshold effects have been observed, where a minimum concentration of this compound is required to achieve its antimitotic effects. Toxicity studies have shown that the therapeutic window for this compound is relatively narrow, necessitating careful dosage optimization .
Metabolic Pathways
This compound is metabolized primarily in the liver, where it undergoes phase I and phase II metabolic reactions. The primary enzymes involved in its metabolism are cytochrome P450 isoforms, particularly CYP3A4/5. These enzymes catalyze the N- and O-dealkylation of this compound, leading to the formation of various metabolites. The metabolic stability of this compound varies between species, with higher clearance rates observed in rat liver microsomes compared to human liver microsomes. This difference in metabolic stability can influence the pharmacokinetics and efficacy of this compound .
Transport and Distribution
This compound is transported and distributed within cells and tissues through passive diffusion and active transport mechanisms. It interacts with various transporters and binding proteins, which facilitate its uptake and distribution. This compound tends to accumulate in tissues with high metabolic activity, such as the liver and kidneys. Its localization within cells is influenced by its lipophilicity and affinity for cellular membranes. The distribution of this compound can also be affected by its binding to plasma proteins, which can modulate its bioavailability and efficacy .
Subcellular Localization
The subcellular localization of this compound is primarily within the cytoplasm, where it interacts with microtubules. It can also localize to the nucleus, where it affects gene expression and cell cycle regulation. The targeting of this compound to specific cellular compartments is influenced by its chemical structure and post-translational modifications. These modifications can include phosphorylation and glycosylation, which can alter the activity and function of this compound. The subcellular localization of this compound is crucial for its antimitotic effects and overall efficacy .
properties
IUPAC Name |
4-[(3,5-dibromo-4-methoxyphenyl)methyl]-2-(methylamino)-1H-imidazo[4,5-d]azepin-5-one | |
---|---|---|
Source | PubChem | |
URL | https://pubchem.ncbi.nlm.nih.gov | |
Description | Data deposited in or computed by PubChem | |
InChI |
InChI=1S/C16H14Br2N4O2/c1-19-16-21-12-3-4-20-15(23)9(13(12)22-16)5-8-6-10(17)14(24-2)11(18)7-8/h3-4,6-7H,5H2,1-2H3,(H2,19,21,22) | |
Source | PubChem | |
URL | https://pubchem.ncbi.nlm.nih.gov | |
Description | Data deposited in or computed by PubChem | |
InChI Key |
KRAXBUZDPZIHER-UHFFFAOYSA-N | |
Source | PubChem | |
URL | https://pubchem.ncbi.nlm.nih.gov | |
Description | Data deposited in or computed by PubChem | |
Canonical SMILES |
CNC1=NC2=C(C(=O)N=CC=C2N1)CC3=CC(=C(C(=C3)Br)OC)Br | |
Source | PubChem | |
URL | https://pubchem.ncbi.nlm.nih.gov | |
Description | Data deposited in or computed by PubChem | |
Molecular Formula |
C16H14Br2N4O2 | |
Source | PubChem | |
URL | https://pubchem.ncbi.nlm.nih.gov | |
Description | Data deposited in or computed by PubChem | |
DSSTOX Substance ID |
DTXSID40458581 | |
Record name | Ceratamine B | |
Source | EPA DSSTox | |
URL | https://comptox.epa.gov/dashboard/DTXSID40458581 | |
Description | DSSTox provides a high quality public chemistry resource for supporting improved predictive toxicology. | |
Molecular Weight |
454.12 g/mol | |
Source | PubChem | |
URL | https://pubchem.ncbi.nlm.nih.gov | |
Description | Data deposited in or computed by PubChem | |
CAS RN |
634151-16-9 | |
Record name | Ceratamine B | |
Source | EPA DSSTox | |
URL | https://comptox.epa.gov/dashboard/DTXSID40458581 | |
Description | DSSTox provides a high quality public chemistry resource for supporting improved predictive toxicology. | |
Retrosynthesis Analysis
AI-Powered Synthesis Planning: Our tool employs the Template_relevance Pistachio, Template_relevance Bkms_metabolic, Template_relevance Pistachio_ringbreaker, Template_relevance Reaxys, Template_relevance Reaxys_biocatalysis model, leveraging a vast database of chemical reactions to predict feasible synthetic routes.
One-Step Synthesis Focus: Specifically designed for one-step synthesis, it provides concise and direct routes for your target compounds, streamlining the synthesis process.
Accurate Predictions: Utilizing the extensive PISTACHIO, BKMS_METABOLIC, PISTACHIO_RINGBREAKER, REAXYS, REAXYS_BIOCATALYSIS database, our tool offers high-accuracy predictions, reflecting the latest in chemical research and data.
Strategy Settings
Precursor scoring | Relevance Heuristic |
---|---|
Min. plausibility | 0.01 |
Model | Template_relevance |
Template Set | Pistachio/Bkms_metabolic/Pistachio_ringbreaker/Reaxys/Reaxys_biocatalysis |
Top-N result to add to graph | 6 |
Feasible Synthetic Routes
Q & A
Q1: What is known about the metabolism of Ceratamine B in humans and rats?
A2: Studies utilizing liver microsomes show that this compound undergoes phase I metabolism in both rats and humans, primarily through N- and O-dealkylations [, ]. While both species exhibit similar metabolic pathways, the major metabolites differ. In rat liver microsomes, the aminoimidazole moiety is the primary site of metabolism, while the dibrominated aromatic ring is the preferred site in human liver microsomes []. Interestingly, this compound demonstrates higher stability in human liver microsomes compared to rat liver microsomes [].
Q2: What is the significance of the structural simplicity of this compound in the context of drug development?
A3: this compound, unlike many other microtubule-stabilizing agents, possesses a remarkably simple structure devoid of chiral centers []. This inherent structural simplicity offers a significant advantage in drug development. It potentially translates to less complex synthesis pathways, easier characterization, and potentially reduced manufacturing costs. Furthermore, this simplicity can be advantageous for structure-activity relationship (SAR) studies, allowing for more straightforward modifications to optimize its pharmacological properties.
Disclaimer and Information on In-Vitro Research Products
Please be aware that all articles and product information presented on BenchChem are intended solely for informational purposes. The products available for purchase on BenchChem are specifically designed for in-vitro studies, which are conducted outside of living organisms. In-vitro studies, derived from the Latin term "in glass," involve experiments performed in controlled laboratory settings using cells or tissues. It is important to note that these products are not categorized as medicines or drugs, and they have not received approval from the FDA for the prevention, treatment, or cure of any medical condition, ailment, or disease. We must emphasize that any form of bodily introduction of these products into humans or animals is strictly prohibited by law. It is essential to adhere to these guidelines to ensure compliance with legal and ethical standards in research and experimentation.