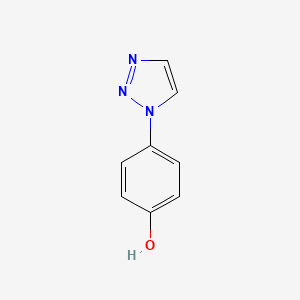
4-(1H-1,2,3-triazol-1-yl)phenol
Overview
Description
4-(1H-1,2,3-triazol-1-yl)phenol is an organic compound that features a phenol group substituted with a 1H-1,2,3-triazole ring. This compound is of significant interest due to its potential applications in various fields, including medicinal chemistry and material science. The presence of both phenolic and triazole functionalities imparts unique chemical properties to this molecule, making it a versatile building block for the synthesis of more complex structures.
Mechanism of Action
Target of Action
The primary target of the compound 4-(1H-1,2,3-triazol-1-yl)phenol is the enzyme Steroid Sulfatase (STS) . STS is a crucial enzyme for steroidogenesis, acting by hydrolyzing inactive steroid sulfates, which are the precursors for the biosynthesis of active estrogens and androgens .
Mode of Action
The compound this compound interacts with its target, STS, by inhibiting its activity . This inhibition prevents the hydrolysis of inactive steroid sulfates, thereby reducing the production of active estrogens and androgens .
Biochemical Pathways
By inhibiting STS, this compound affects the steroidogenesis pathway . This results in a decrease in the levels of active estrogens and androgens, which are crucial for the growth and proliferation of certain types of cancer cells .
Pharmacokinetics
The presence of the 1,2,4-triazole moiety in the compound can influence its dipole moment, polarity, solubility, lipophilicity, and hydrogen bonding capacity . These properties can potentially impact the compound’s Absorption, Distribution, Metabolism, and Excretion (ADME) properties, and thus its bioavailability.
Result of Action
The inhibition of STS by this compound leads to a decrease in the levels of active estrogens and androgens . This can result in the inhibition of the growth and proliferation of hormone-dependent cancer cells . For instance, it has been shown to have potent inhibitory activities against MCF-7 and HCT-116 cancer cell lines .
Biochemical Analysis
Biochemical Properties
In biochemical reactions, 4-(1H-1,2,3-triazol-1-yl)phenol has been shown to interact with Heat shock protein 90 (HSP90), a molecular chaperone . HSP90 is essential for cell viability, ATP-dependent folding, and assembling of its client proteins . The interaction between this compound and HSP90 has been characterized by significant binding affinity .
Cellular Effects
The effects of this compound on cells are primarily mediated through its interaction with HSP90. By binding to HSP90, this compound can influence cell function, including impacts on cell signaling pathways, gene expression, and cellular metabolism .
Molecular Mechanism
At the molecular level, this compound exerts its effects through binding interactions with HSP90. This binding can lead to changes in gene expression and can influence the activity of HSP90’s client proteins .
Temporal Effects in Laboratory Settings
Preliminary studies have shown that certain compounds with this structure exhibit significant binding affinity to HSP90 .
Metabolic Pathways
Given its interaction with HSP90, it is likely that this compound could influence the metabolic pathways associated with this protein .
Preparation Methods
Synthetic Routes and Reaction Conditions
The synthesis of 4-(1H-1,2,3-triazol-1-yl)phenol typically involves a copper-catalyzed azide-alkyne cycloaddition (CuAAC), also known as the “click” reaction. This method is favored for its high efficiency and selectivity. The general procedure includes the following steps:
Preparation of the Azide: The starting material, 4-iodophenol, is converted to 4-azidophenol using sodium azide in the presence of a copper catalyst.
Cycloaddition Reaction: The azide is then reacted with an alkyne (such as propargyl alcohol) in the presence of a copper(I) catalyst to form the 1,2,3-triazole ring.
The reaction conditions typically involve the use of solvents like dimethyl sulfoxide (DMSO) or water, and the reaction is carried out at room temperature or slightly elevated temperatures.
Industrial Production Methods
Industrial production of this compound follows similar synthetic routes but on a larger scale. The process is optimized for higher yields and purity, often involving continuous flow reactors and automated systems to ensure consistent product quality.
Chemical Reactions Analysis
Types of Reactions
4-(1H-1,2,3-triazol-1-yl)phenol undergoes various chemical reactions, including:
Oxidation: The phenolic group can be oxidized to form quinones or other oxidized derivatives.
Reduction: The triazole ring can be reduced under specific conditions to form dihydrotriazoles.
Substitution: The phenolic hydroxyl group can participate in nucleophilic substitution reactions, forming ethers or esters.
Common Reagents and Conditions
Oxidation: Reagents like potassium permanganate (KMnO4) or hydrogen peroxide (H2O2) are commonly used.
Reduction: Catalytic hydrogenation using palladium on carbon (Pd/C) is a typical method.
Substitution: Alkyl halides or acyl chlorides are used in the presence of a base like sodium hydroxide (NaOH) or potassium carbonate (K2CO3).
Major Products
Oxidation: Quinones or hydroquinones.
Reduction: Dihydrotriazoles.
Substitution: Ethers or esters of this compound.
Scientific Research Applications
4-(1H-1,2,3-triazol-1-yl)phenol has a wide range of applications in scientific research:
Chemistry: Used as a building block for the synthesis of more complex molecules, including polymers and dendrimers.
Biology: Acts as a ligand in the study of enzyme inhibition and protein interactions.
Industry: Utilized in the development of new materials with unique properties, such as enhanced thermal stability and conductivity.
Comparison with Similar Compounds
Similar Compounds
- 4-(1H-1,2,4-triazol-1-yl)phenol
- 4-(1H-imidazol-1-yl)phenol
- 4-(1H-pyrazol-1-yl)phenol
Comparison
Compared to similar compounds, 4-(1H-1,2,3-triazol-1-yl)phenol exhibits unique properties due to the presence of the 1,2,3-triazole ring. This ring system provides enhanced stability and specific binding interactions with biological targets, making it a more effective inhibitor in certain biochemical pathways .
Properties
IUPAC Name |
4-(triazol-1-yl)phenol | |
---|---|---|
Details | Computed by Lexichem TK 2.7.0 (PubChem release 2021.05.07) | |
Source | PubChem | |
URL | https://pubchem.ncbi.nlm.nih.gov | |
Description | Data deposited in or computed by PubChem | |
InChI |
InChI=1S/C8H7N3O/c12-8-3-1-7(2-4-8)11-6-5-9-10-11/h1-6,12H | |
Details | Computed by InChI 1.0.6 (PubChem release 2021.05.07) | |
Source | PubChem | |
URL | https://pubchem.ncbi.nlm.nih.gov | |
Description | Data deposited in or computed by PubChem | |
InChI Key |
RSZRMXYZBCUXAL-UHFFFAOYSA-N | |
Details | Computed by InChI 1.0.6 (PubChem release 2021.05.07) | |
Source | PubChem | |
URL | https://pubchem.ncbi.nlm.nih.gov | |
Description | Data deposited in or computed by PubChem | |
Canonical SMILES |
C1=CC(=CC=C1N2C=CN=N2)O | |
Details | Computed by OEChem 2.3.0 (PubChem release 2021.05.07) | |
Source | PubChem | |
URL | https://pubchem.ncbi.nlm.nih.gov | |
Description | Data deposited in or computed by PubChem | |
Molecular Formula |
C8H7N3O | |
Details | Computed by PubChem 2.1 (PubChem release 2021.05.07) | |
Source | PubChem | |
URL | https://pubchem.ncbi.nlm.nih.gov | |
Description | Data deposited in or computed by PubChem | |
Molecular Weight |
161.16 g/mol | |
Details | Computed by PubChem 2.1 (PubChem release 2021.05.07) | |
Source | PubChem | |
URL | https://pubchem.ncbi.nlm.nih.gov | |
Description | Data deposited in or computed by PubChem | |
CAS No. |
68535-50-2 | |
Record name | 4-(1H-1,2,3-triazol-1-yl)phenol | |
Source | European Chemicals Agency (ECHA) | |
URL | https://echa.europa.eu/information-on-chemicals | |
Description | The European Chemicals Agency (ECHA) is an agency of the European Union which is the driving force among regulatory authorities in implementing the EU's groundbreaking chemicals legislation for the benefit of human health and the environment as well as for innovation and competitiveness. | |
Explanation | Use of the information, documents and data from the ECHA website is subject to the terms and conditions of this Legal Notice, and subject to other binding limitations provided for under applicable law, the information, documents and data made available on the ECHA website may be reproduced, distributed and/or used, totally or in part, for non-commercial purposes provided that ECHA is acknowledged as the source: "Source: European Chemicals Agency, http://echa.europa.eu/". Such acknowledgement must be included in each copy of the material. ECHA permits and encourages organisations and individuals to create links to the ECHA website under the following cumulative conditions: Links can only be made to webpages that provide a link to the Legal Notice page. | |
Synthesis routes and methods
Procedure details
Disclaimer and Information on In-Vitro Research Products
Please be aware that all articles and product information presented on BenchChem are intended solely for informational purposes. The products available for purchase on BenchChem are specifically designed for in-vitro studies, which are conducted outside of living organisms. In-vitro studies, derived from the Latin term "in glass," involve experiments performed in controlled laboratory settings using cells or tissues. It is important to note that these products are not categorized as medicines or drugs, and they have not received approval from the FDA for the prevention, treatment, or cure of any medical condition, ailment, or disease. We must emphasize that any form of bodily introduction of these products into humans or animals is strictly prohibited by law. It is essential to adhere to these guidelines to ensure compliance with legal and ethical standards in research and experimentation.