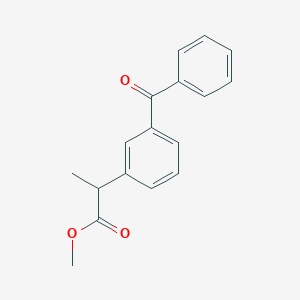
Ketoprofen methyl ester
Overview
Description
Ketoprofen methyl ester is a compound with the molecular formula C17H16O3 . It is also known by other names such as Methyl 2- (3-benzoylphenyl)propanoate and Methyl 2- (3-benzoylphenyl)propionate . The molecular weight of this compound is 268.31 g/mol .
Synthesis Analysis
Ester prodrugs of ketoprofen, including ketoprofen methyl ester, have been synthesized and characterized by IR, 1H NMR, and mass spectral data . The synthesis process involves the dicyclohexyl carbodiimide (DCC) coupling method .Molecular Structure Analysis
The IUPAC name for Ketoprofen methyl ester is methyl 2- (3-benzoylphenyl)propanoate . The InChI and Canonical SMILES for this compound are also available .Chemical Reactions Analysis
Ester prodrugs of ketoprofen are chemically stable and possess increased lipophilicity compared to their parent compounds . They are converted to the active drugs in vivo .Physical And Chemical Properties Analysis
Ketoprofen methyl ester has a molecular weight of 268.31 g/mol . It has a XLogP3 value of 3.4, indicating its lipophilicity . It has 0 hydrogen bond donors and 3 hydrogen bond acceptors . It also has 5 rotatable bonds .Scientific Research Applications
Imaging in Neuroinflammation
Ketoprofen methyl ester has been used in the synthesis of 18F-labeled Ketoprofen Methyl Esters for Cyclooxygenase-1 Imaging in Neuroinflammation . This is a potentially powerful tool for assessing the inflammatory response to injury, infection, and disease .
Reduction of Hepatotoxicity and Gastrointestinal Irritation
Ketoprofen prodrugs have been synthesized with the aim to reduce its hepatotoxicity and gastrointestinal irritation . The research aimed to demonstrate their potentiality for oral treatment to treat chronic inflammation .
Topical Delivery of Ketoprofen
The nanoemulsion formulated using Palm Oil Esters (POEs) has shown great potential for topical delivery of ketoprofen . The in vitro release profile shows a sufficient percentage of drugs released through the methyl acetate cellulose membrane .
Analysis of Basic Drugs in Horse Urine Samples
An unexpected formation of ketoprofen methyl ester (KME) was observed during the routine alkaline liquid–liquid extraction (LLE) process for analyzing basic drugs in horse urine samples using GC–MS analysis .
Anti-proteolytic and Lysosomal Membrane Stabilization Potentials
The prodrugs of ketoprofen were found to exhibit not only remarkable in vitro anti-proteolytic and lysosomal membrane stabilization potentials , but also significant efficiency to alleviate pain induced by inflammation, as well as central and peripheral stimulus in mice model in vivo .
Ex Vivo Intestinal Permeation Enhancement
The ex vivo intestinal permeation enhancement ratio of the prodrugs was statistically significant compared to ketoprofen . This suggests that these prodrugs could potentially improve the bioavailability of ketoprofen.
Future Directions
Research is ongoing to understand the role of compounds like Ketoprofen methyl ester in neuroinflammation and neurodegenerative disorders . One such analog, 11 C-ketoprofen-methyl ester, displayed promising cyclooxygenase-1 selectivity and brain uptake in rats with systemic inflammation . This suggests potential future directions for the use of Ketoprofen methyl ester in medical imaging and treatment of neuroinflammatory conditions .
Mechanism of Action
Target of Action
Ketoprofen methyl ester primarily targets Cyclooxygenase-1 (COX-1) . COX-1 is a rate-limiting enzyme in the synthesis of pro-inflammatory prostanoids from arachidonic acid . It plays a crucial role in the inflammatory response to injury, infection, and disease .
Mode of Action
Ketoprofen methyl ester acts as a prodrug . It penetrates the blood-brain barrier and undergoes hydrolysis to its pharmacologically active acid form . The anti-inflammatory effects of ketoprofen are believed to be due to the inhibition of COX-1 . This inhibition results in a decrease in the synthesis of pro-inflammatory prostanoids from arachidonic acid .
Biochemical Pathways
The primary biochemical pathway affected by ketoprofen methyl ester is the arachidonic acid pathway . By inhibiting COX-1, ketoprofen methyl ester reduces the production of pro-inflammatory prostanoids, which are key mediators of inflammation and pain .
Pharmacokinetics
Ketoprofen methyl ester is a prodrug that is designed to improve the bioavailability of ketoprofen . It crosses the blood-brain barrier and is subsequently hydrolyzed to its active form . The duodenum and jejunum regions of the intestine metabolize the ester to form active drugs and aid in absorption .
Result of Action
The result of ketoprofen methyl ester’s action is a reduction in inflammation and pain. In vivo imaging studies have shown that it can detect COX-1 activation in models of neuroinflammation and neurodegenerative disorders . Moreover, PET images revealed a high accumulation of ketoprofen methyl ester in the inflamed regions in rat brain .
Action Environment
The action of ketoprofen methyl ester can be influenced by various environmental factors. For instance, the stability of the compound in plasma can vary between different species . Additionally, the hydrolytic activities in commercially purchased and freshly collected plasma may be different and species-dependent . Esterase inhibitors also have different effects on preventing hydrolysis of the ester-containing drugs in the plasma of different species .
properties
IUPAC Name |
methyl 2-(3-benzoylphenyl)propanoate | |
---|---|---|
Source | PubChem | |
URL | https://pubchem.ncbi.nlm.nih.gov | |
Description | Data deposited in or computed by PubChem | |
InChI |
InChI=1S/C17H16O3/c1-12(17(19)20-2)14-9-6-10-15(11-14)16(18)13-7-4-3-5-8-13/h3-12H,1-2H3 | |
Source | PubChem | |
URL | https://pubchem.ncbi.nlm.nih.gov | |
Description | Data deposited in or computed by PubChem | |
InChI Key |
BIOCOYIPJQMGTN-UHFFFAOYSA-N | |
Source | PubChem | |
URL | https://pubchem.ncbi.nlm.nih.gov | |
Description | Data deposited in or computed by PubChem | |
Canonical SMILES |
CC(C1=CC(=CC=C1)C(=O)C2=CC=CC=C2)C(=O)OC | |
Source | PubChem | |
URL | https://pubchem.ncbi.nlm.nih.gov | |
Description | Data deposited in or computed by PubChem | |
Molecular Formula |
C17H16O3 | |
Source | PubChem | |
URL | https://pubchem.ncbi.nlm.nih.gov | |
Description | Data deposited in or computed by PubChem | |
DSSTOX Substance ID |
DTXSID901346303 | |
Record name | Ketoprofen methyl ester | |
Source | EPA DSSTox | |
URL | https://comptox.epa.gov/dashboard/DTXSID901346303 | |
Description | DSSTox provides a high quality public chemistry resource for supporting improved predictive toxicology. | |
Molecular Weight |
268.31 g/mol | |
Source | PubChem | |
URL | https://pubchem.ncbi.nlm.nih.gov | |
Description | Data deposited in or computed by PubChem | |
Product Name |
Ketoprofen methyl ester | |
CAS RN |
47087-07-0 | |
Record name | Ketoprofen methyl ester | |
Source | EPA DSSTox | |
URL | https://comptox.epa.gov/dashboard/DTXSID901346303 | |
Description | DSSTox provides a high quality public chemistry resource for supporting improved predictive toxicology. | |
Synthesis routes and methods I
Procedure details
Synthesis routes and methods II
Procedure details
Synthesis routes and methods III
Procedure details
Synthesis routes and methods IV
Procedure details
Retrosynthesis Analysis
AI-Powered Synthesis Planning: Our tool employs the Template_relevance Pistachio, Template_relevance Bkms_metabolic, Template_relevance Pistachio_ringbreaker, Template_relevance Reaxys, Template_relevance Reaxys_biocatalysis model, leveraging a vast database of chemical reactions to predict feasible synthetic routes.
One-Step Synthesis Focus: Specifically designed for one-step synthesis, it provides concise and direct routes for your target compounds, streamlining the synthesis process.
Accurate Predictions: Utilizing the extensive PISTACHIO, BKMS_METABOLIC, PISTACHIO_RINGBREAKER, REAXYS, REAXYS_BIOCATALYSIS database, our tool offers high-accuracy predictions, reflecting the latest in chemical research and data.
Strategy Settings
Precursor scoring | Relevance Heuristic |
---|---|
Min. plausibility | 0.01 |
Model | Template_relevance |
Template Set | Pistachio/Bkms_metabolic/Pistachio_ringbreaker/Reaxys/Reaxys_biocatalysis |
Top-N result to add to graph | 6 |
Feasible Synthetic Routes
Disclaimer and Information on In-Vitro Research Products
Please be aware that all articles and product information presented on BenchChem are intended solely for informational purposes. The products available for purchase on BenchChem are specifically designed for in-vitro studies, which are conducted outside of living organisms. In-vitro studies, derived from the Latin term "in glass," involve experiments performed in controlled laboratory settings using cells or tissues. It is important to note that these products are not categorized as medicines or drugs, and they have not received approval from the FDA for the prevention, treatment, or cure of any medical condition, ailment, or disease. We must emphasize that any form of bodily introduction of these products into humans or animals is strictly prohibited by law. It is essential to adhere to these guidelines to ensure compliance with legal and ethical standards in research and experimentation.