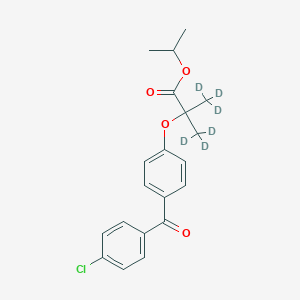
Fenofibrate-d6
Overview
Description
PTP Inhibitor I is a compound known for its ability to inhibit protein tyrosine phosphatases (PTPs). Protein tyrosine phosphatases are enzymes that remove phosphate groups from phosphorylated tyrosine residues on proteins, playing a crucial role in regulating cellular processes such as cell growth, differentiation, and metabolism. PTP Inhibitor I has been studied for its potential therapeutic applications in various diseases, including diabetes, cancer, and neurodegenerative disorders .
Mechanism of Action
Target of Action
Fenofibrate primarily targets the Peroxisome Proliferator-Activated Receptor Alpha (PPARα) . PPARα is a nuclear receptor that plays a crucial role in lipid, glucose, and amino acid homeostasis . It also has a significant role in the regulation of genes involved in inflammation, angiogenesis, and cell apoptosis .
Mode of Action
Fenofibrate is rapidly converted to fenofibric acid in vivo, which binds to PPARα and forms a heterodimer complex with retinoid X receptor (RXR) . This binding activates PPARα, leading to the transcription of various genes that regulate lipid metabolism, inflammation, angiogenesis, and cell apoptosis . The activation of PPARα also increases lipolysis and reduces apoprotein C-III .
Biochemical Pathways
Fenofibrate affects several biochemical pathways. It enhances the PPARα/PGC-1α signaling pathway , promoting mitochondrial β-oxidation, reducing oxidative stress damage, and lipid accumulation in the liver . It also significantly enhances the biosynthesis of unsaturated fatty acids, glycerophospholipid metabolism, and pyrimidine metabolism, while reducing glyoxylate and dicarboxylate metabolism, tyrosine metabolism, tryptophan metabolism, and nonribosomal peptide structures .
Pharmacokinetics
Fenofibrate is known to have a lipid-lowering effect. It is readily and entirely transformed into fenofibric acid, which can be partially metabolized into a reduced derivative . The absorption of fenofibrate is significantly increased after food consumption, which is believed to be due to the increased gastric emptying rate and the increased calories in the duodenum .
Result of Action
The activation of PPARα by fenofibrate leads to a range of molecular and cellular effects. It improves the survival of retinal endothelial and pigment epithelial cells under diabetic conditions . It also inhibits the proliferation of gliomas . In the liver, it promotes mitochondrial β-oxidation, reduces oxidative stress damage, and lipid accumulation .
Action Environment
Environmental factors, such as diet, can influence the action of fenofibrate. For instance, a high-fat diet can significantly alter the composition and metabolic pathways of gut microbiota, which in turn can affect the action of fenofibrate . Fenofibrate treatment significantly reduces serum lipid levels in hyperlipidemia hamsters fed a high-fat diet .
Biochemical Analysis
Biochemical Properties
Fenofibrate-d6 interacts with PPARα, a key regulator of lipid metabolism . It has EC50 values of 18 and 30 μM for mouse and human receptors, respectively . It is selective for PPARα over PPARγ and lacks activity at mouse and human PPARδ at a concentration of 100 μM .
Cellular Effects
This compound has been shown to significantly reduce serum lipid levels in hyperlipidemia hamsters . It also increases the levels of beneficial bacterial species associated with health, including Bacteroides ovatus, Bifidobacterium animalis, Bacteroides intestinalis, Allobaculum stercoricanis, Lactobacillus reuteri, and Bacteroides acidifaciens .
Molecular Mechanism
This compound exerts its effects at the molecular level by binding to PPARα, leading to the activation of this receptor . This activation results in the modulation of genes involved in lipid metabolism, leading to decreased levels of triglycerides and low-density lipoprotein cholesterol, and increased levels of high-density lipoprotein cholesterol .
Temporal Effects in Laboratory Settings
In laboratory settings, this compound has been shown to have long-term effects on cellular function. For instance, it has been observed to significantly reduce the progression of diabetic retinopathy and other microvascular endpoints in patients with type 2 diabetes over a mean 4.03-year follow-up period .
Dosage Effects in Animal Models
In animal models, the effects of this compound vary with different dosages. For example, in a study involving hamsters fed a high-fat diet, fenofibrate treatments significantly reduced the serum lipid levels in hyperlipidemia hamsters . The group treated with fenofibrate exhibited higher levels of beneficial bacterial species associated with health .
Metabolic Pathways
This compound is involved in the PPARα pathway, which plays a crucial role in lipid metabolism . It enhances the biosynthesis of unsaturated fatty acids, glycerophospholipid metabolism, and pyrimidine metabolism, while reducing glyoxylate and dicarboxylate metabolism, tyrosine metabolism, tryptophan metabolism, and nonribosomal peptide structures .
Transport and Distribution
This compound is absorbed from the gastrointestinal tract and is principally hydrolyzed by the CYP3A4 isozyme to its active and major metabolite, fenofibric acid . The absorption rate of this compound is significantly affected by the fat content of ingested food .
Subcellular Localization
The subcellular localization of this compound is primarily within the liver, heart, muscle, and kidney, where it augments fatty acid catabolism . It is also found in the cells of the gastrointestinal tract where it is absorbed .
Preparation Methods
Synthetic Routes and Reaction Conditions
The synthesis of PTP Inhibitor I involves several steps, starting with the preparation of key intermediates. The synthetic route typically includes the following steps:
Formation of the Core Structure: The core structure of PTP Inhibitor I is synthesized through a series of condensation and cyclization reactions.
Functional Group Modifications: Various functional groups are introduced to the core structure through substitution reactions, using reagents such as halides and amines.
Purification: The final product is purified using techniques such as recrystallization and chromatography to obtain a high-purity compound.
Industrial Production Methods
Industrial production of PTP Inhibitor I follows similar synthetic routes but on a larger scale. The process involves optimizing reaction conditions to maximize yield and minimize impurities. Techniques such as continuous flow reactors and automated synthesis platforms are employed to enhance efficiency and scalability .
Chemical Reactions Analysis
Types of Reactions
PTP Inhibitor I undergoes various chemical reactions, including:
Oxidation: The compound can be oxidized using oxidizing agents such as hydrogen peroxide or potassium permanganate.
Reduction: Reduction reactions can be carried out using reducing agents like sodium borohydride or lithium aluminum hydride.
Substitution: Substitution reactions involve replacing functional groups with other groups, using reagents such as halides or amines.
Common Reagents and Conditions
Oxidation: Hydrogen peroxide, potassium permanganate; typically carried out in aqueous or organic solvents.
Reduction: Sodium borohydride, lithium aluminum hydride; reactions are performed under inert atmosphere conditions.
Substitution: Halides, amines; reactions are conducted in polar or non-polar solvents, depending on the specific substitution.
Major Products Formed
The major products formed from these reactions include various derivatives of PTP Inhibitor I with modified functional groups, which can be further studied for their biological activities .
Scientific Research Applications
PTP Inhibitor I has a wide range of scientific research applications:
Chemistry: Used as a tool to study protein phosphorylation and dephosphorylation processes.
Biology: Investigated for its role in regulating cellular signaling pathways and its potential as a therapeutic agent.
Medicine: Explored for its potential to treat diseases such as diabetes, cancer, and neurodegenerative disorders by modulating PTP activity.
Industry: Utilized in the development of new drugs and therapeutic agents targeting PTPs
Comparison with Similar Compounds
Similar Compounds
PTP1B Inhibitors: Compounds that specifically inhibit protein tyrosine phosphatase 1B, such as ABBV-CLS-484 and Compound-182.
Vanadium Compounds: Vanadium-based inhibitors that mimic phosphate and inhibit a broad range of PTPs.
Uniqueness
PTP Inhibitor I is unique in its ability to selectively inhibit multiple PTPs, making it a versatile tool for studying various cellular processes and potential therapeutic applications. Unlike some other inhibitors, PTP Inhibitor I has shown efficacy in modulating multiple signaling pathways, providing a broader scope of research and therapeutic potential .
Properties
IUPAC Name |
propan-2-yl 2-[4-(4-chlorobenzoyl)phenoxy]-3,3,3-trideuterio-2-(trideuteriomethyl)propanoate | |
---|---|---|
Source | PubChem | |
URL | https://pubchem.ncbi.nlm.nih.gov | |
Description | Data deposited in or computed by PubChem | |
InChI |
InChI=1S/C20H21ClO4/c1-13(2)24-19(23)20(3,4)25-17-11-7-15(8-12-17)18(22)14-5-9-16(21)10-6-14/h5-13H,1-4H3/i3D3,4D3 | |
Source | PubChem | |
URL | https://pubchem.ncbi.nlm.nih.gov | |
Description | Data deposited in or computed by PubChem | |
InChI Key |
YMTINGFKWWXKFG-LIJFRPJRSA-N | |
Source | PubChem | |
URL | https://pubchem.ncbi.nlm.nih.gov | |
Description | Data deposited in or computed by PubChem | |
Canonical SMILES |
CC(C)OC(=O)C(C)(C)OC1=CC=C(C=C1)C(=O)C2=CC=C(C=C2)Cl | |
Source | PubChem | |
URL | https://pubchem.ncbi.nlm.nih.gov | |
Description | Data deposited in or computed by PubChem | |
Isomeric SMILES |
[2H]C([2H])([2H])C(C(=O)OC(C)C)(C([2H])([2H])[2H])OC1=CC=C(C=C1)C(=O)C2=CC=C(C=C2)Cl | |
Source | PubChem | |
URL | https://pubchem.ncbi.nlm.nih.gov | |
Description | Data deposited in or computed by PubChem | |
Molecular Formula |
C20H21ClO4 | |
Source | PubChem | |
URL | https://pubchem.ncbi.nlm.nih.gov | |
Description | Data deposited in or computed by PubChem | |
DSSTOX Substance ID |
DTXSID50648850 | |
Record name | Propan-2-yl 2-[4-(4-chlorobenzoyl)phenoxy]-2-(~2~H_3_)methyl(~2~H_3_)propanoate | |
Source | EPA DSSTox | |
URL | https://comptox.epa.gov/dashboard/DTXSID50648850 | |
Description | DSSTox provides a high quality public chemistry resource for supporting improved predictive toxicology. | |
Molecular Weight |
366.9 g/mol | |
Source | PubChem | |
URL | https://pubchem.ncbi.nlm.nih.gov | |
Description | Data deposited in or computed by PubChem | |
CAS No. |
1092484-56-4 | |
Record name | Propan-2-yl 2-[4-(4-chlorobenzoyl)phenoxy]-2-(~2~H_3_)methyl(~2~H_3_)propanoate | |
Source | EPA DSSTox | |
URL | https://comptox.epa.gov/dashboard/DTXSID50648850 | |
Description | DSSTox provides a high quality public chemistry resource for supporting improved predictive toxicology. | |
Synthesis routes and methods I
Procedure details
Synthesis routes and methods II
Procedure details
Synthesis routes and methods III
Procedure details
Synthesis routes and methods IV
Procedure details
Retrosynthesis Analysis
AI-Powered Synthesis Planning: Our tool employs the Template_relevance Pistachio, Template_relevance Bkms_metabolic, Template_relevance Pistachio_ringbreaker, Template_relevance Reaxys, Template_relevance Reaxys_biocatalysis model, leveraging a vast database of chemical reactions to predict feasible synthetic routes.
One-Step Synthesis Focus: Specifically designed for one-step synthesis, it provides concise and direct routes for your target compounds, streamlining the synthesis process.
Accurate Predictions: Utilizing the extensive PISTACHIO, BKMS_METABOLIC, PISTACHIO_RINGBREAKER, REAXYS, REAXYS_BIOCATALYSIS database, our tool offers high-accuracy predictions, reflecting the latest in chemical research and data.
Strategy Settings
Precursor scoring | Relevance Heuristic |
---|---|
Min. plausibility | 0.01 |
Model | Template_relevance |
Template Set | Pistachio/Bkms_metabolic/Pistachio_ringbreaker/Reaxys/Reaxys_biocatalysis |
Top-N result to add to graph | 6 |
Feasible Synthetic Routes
Disclaimer and Information on In-Vitro Research Products
Please be aware that all articles and product information presented on BenchChem are intended solely for informational purposes. The products available for purchase on BenchChem are specifically designed for in-vitro studies, which are conducted outside of living organisms. In-vitro studies, derived from the Latin term "in glass," involve experiments performed in controlled laboratory settings using cells or tissues. It is important to note that these products are not categorized as medicines or drugs, and they have not received approval from the FDA for the prevention, treatment, or cure of any medical condition, ailment, or disease. We must emphasize that any form of bodily introduction of these products into humans or animals is strictly prohibited by law. It is essential to adhere to these guidelines to ensure compliance with legal and ethical standards in research and experimentation.