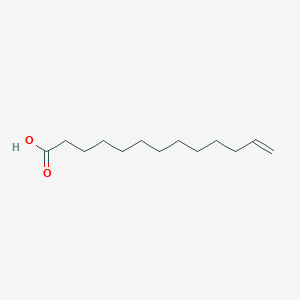
12-Tridecenoic acid
Overview
Description
12-Tridecenoic Acid: is a monounsaturated fatty acid with the molecular formula C13H24O2 . It is characterized by a long hydrocarbon chain with a single double bond located at the twelfth carbon atom from the carboxyl end. This compound has garnered interest in various fields, such as lipidomics and biochemistry, due to its incorporation into complex lipid structures and the resulting effects on membrane fluidity and function .
Mechanism of Action
Target of Action
12-Tridecenoic acid is a monounsaturated fatty acid . It is primarily targeted at complex lipid structures in the body . These lipid structures play a crucial role in maintaining the fluidity and function of cell membranes .
Mode of Action
The compound interacts with its targets by incorporating itself into these complex lipid structures . This incorporation alters the physical properties of the cell membranes, such as their fluidity and function .
Biochemical Pathways
The primary biochemical pathway affected by this compound is lipid metabolism . As a long-chain fatty acid , it is involved in various metabolic processes. The downstream effects of this pathway can influence the overall lipid profile of the organism, affecting energy production and storage, hormone regulation, and cellular communication.
Result of Action
The primary molecular effect of this compound’s action is the alteration of membrane fluidity and function . This can have various cellular effects, depending on the specific roles of the affected cells. For example, changes in membrane fluidity can influence the activity of membrane-bound enzymes and receptors, potentially impacting cellular signaling and metabolic processes.
Biochemical Analysis
Biochemical Properties
Studies involving 12-Tridecenoic acid focus on its incorporation into complex lipid structures and the resulting effects on membrane fluidity and function . It is also explored in metabolic pathway analyses, where it is investigated for its biosynthesis and degradation, as well as its role in cellular signaling mechanisms . This compound is in the study of enzyme specificity and function, particularly with enzymes that are involved in fatty acid metabolism, such as desaturases and elongases .
Cellular Effects
It is known that it can influence cell function, including any impact on cell signaling pathways, gene expression, and cellular metabolism .
Molecular Mechanism
It is known to exert its effects at the molecular level, including any binding interactions with biomolecules, enzyme inhibition or activation, and changes in gene expression .
Temporal Effects in Laboratory Settings
It is known that it has a role in the stability, degradation, and any long-term effects on cellular function observed in in vitro or in vivo studies .
Dosage Effects in Animal Models
The effects of this compound vary with different dosages in animal models . This includes any threshold effects observed in these studies, as well as any toxic or adverse effects at high doses .
Metabolic Pathways
This compound is involved in various metabolic pathways . This includes any enzymes or cofactors that it interacts with. It also includes any effects on metabolic flux or metabolite levels .
Transport and Distribution
It is known that it can interact with various transporters or binding proteins .
Subcellular Localization
It is known that it can be directed to specific compartments or organelles .
Preparation Methods
Synthetic Routes and Reaction Conditions: 12-Tridecenoic Acid can be synthesized through various methods. One common approach involves the hydrolysis of esters derived from the corresponding unsaturated alcohols. The reaction typically involves the use of strong acids or bases to catalyze the hydrolysis process, followed by purification steps to isolate the desired acid .
Industrial Production Methods: In industrial settings, this compound is often produced through the oxidation of long-chain hydrocarbons . This process involves the controlled oxidation of hydrocarbons using oxygen or other oxidizing agents, followed by purification and isolation of the desired product .
Chemical Reactions Analysis
Types of Reactions: 12-Tridecenoic Acid undergoes various chemical reactions, including:
Reduction: The carboxyl group can be reduced to an alcohol using reducing agents like lithium aluminum hydride.
Substitution: The hydrogen atoms on the hydrocarbon chain can be substituted with halogens or other functional groups using appropriate reagents.
Common Reagents and Conditions:
Oxidation: Potassium permanganate, osmium tetroxide, and hydrogen peroxide.
Reduction: Lithium aluminum hydride, sodium borohydride.
Substitution: Halogens (chlorine, bromine), organometallic reagents.
Major Products Formed:
Epoxides and diols: from oxidation reactions.
Alcohols: from reduction reactions.
Halogenated derivatives: from substitution reactions.
Scientific Research Applications
Chemistry: 12-Tridecenoic Acid is used in the synthesis of complex lipid structures and as a precursor for various chemical reactions. Its unique structure makes it valuable in studying the properties of monounsaturated fatty acids .
Biology: In biological research, this compound is incorporated into lipid membranes to study its effects on membrane fluidity and function. It is also used in studies involving lipid metabolism and signaling pathways .
Medicine: this compound has shown potential in medical research due to its antimicrobial properties . It has been found to be active against certain bacterial strains, making it a candidate for developing new antimicrobial agents .
Industry: In industrial applications, this compound is used as a surfactant and emulsifying agent . Its ability to modify surface tension makes it valuable in the formulation of various products, including cosmetics and pharmaceuticals .
Comparison with Similar Compounds
Oleic Acid: Another monounsaturated fatty acid with a double bond at the ninth carbon atom.
Linoleic Acid: A polyunsaturated fatty acid with two double bonds at the ninth and twelfth carbon atoms.
Palmitoleic Acid: A monounsaturated fatty acid with a double bond at the seventh carbon atom.
Uniqueness: 12-Tridecenoic Acid is unique due to its specific double bond position at the twelfth carbon atom, which imparts distinct chemical and physical properties. This unique structure allows it to interact differently with lipid membranes and enzymes compared to other similar fatty acids .
Biological Activity
12-Tridecenoic acid, a medium-chain fatty acid, has garnered attention for its diverse biological activities, particularly in metabolic regulation and potential therapeutic applications. This article explores its biological activity through various studies and findings.
This compound, also known as tridecenoic acid, is an unsaturated fatty acid with the formula . Its structure features a double bond between the 12th and 13th carbon atoms in the chain. This unique configuration influences its biological functions, including its role in lipid metabolism and cellular signaling.
1. Metabolic Effects
Recent research has highlighted the role of this compound in lipid metabolism, particularly in the context of non-alcoholic steatohepatitis (NASH). A study demonstrated that treatment with this compound significantly increased triglyceride (TG) levels in liver cell lines (AML12 and HepG2) at concentrations of 50 μM and 100 μM . This effect is attributed to its influence on key metabolic pathways:
- Acetyl-CoA Carboxylase Alpha (ACC) : this compound promotes ACC expression, which is involved in fatty acid synthesis.
- Carnitine Palmitoyltransferase 1A (CPT1A) : It inhibits CPT1A expression, leading to reduced fatty acid oxidation.
These actions contribute to the aggravation of hepatic steatosis, highlighting the compound's potential role as a metabolic disruptor in conditions such as NASH .
2. Antimicrobial Activity
This compound has been evaluated for its antimicrobial properties. A study on various fatty acids indicated that certain unsaturated fatty acids exhibit significant antimicrobial activity against pathogens like Staphylococcus aureus. The structure-activity relationship suggests that the presence of double bonds enhances antimicrobial efficacy .
3. Antitumor Potential
Historical studies have also pointed to the antitumor potential of related compounds derived from tridecenoic acids. For instance, derivatives such as 11-oxo-12-tridecenoic acid have shown promise as antitumor agents . This suggests that while this compound itself may not be directly studied for antitumor effects, its derivatives could provide insights into potential therapeutic applications.
Study on Dietary Fatty Acids and Colorectal Cancer Risk
A comprehensive study examined dietary patterns of fatty acids, including odd-chain fatty acids like this compound, and their association with colorectal cancer (CRC) risk. The findings indicated that higher intakes of odd-chain fatty acids were inversely associated with CRC risk, suggesting a protective effect .
Fatty Acid Type | Adjusted Odds Ratio (aOR) | Confidence Interval (CI) |
---|---|---|
Medium-chain SFAs | 0.34 | 0.27–0.42 |
Highly unsaturated fatty acids | 0.73 | 0.60–0.88 |
Odd-chain fatty acids | 0.69 | 0.57–0.83 |
This data underscores the importance of dietary sources of medium and odd-chain fatty acids in cancer prevention strategies.
Properties
IUPAC Name |
tridec-12-enoic acid | |
---|---|---|
Source | PubChem | |
URL | https://pubchem.ncbi.nlm.nih.gov | |
Description | Data deposited in or computed by PubChem | |
InChI |
InChI=1S/C13H24O2/c1-2-3-4-5-6-7-8-9-10-11-12-13(14)15/h2H,1,3-12H2,(H,14,15) | |
Source | PubChem | |
URL | https://pubchem.ncbi.nlm.nih.gov | |
Description | Data deposited in or computed by PubChem | |
InChI Key |
PARCICAYFORNPH-UHFFFAOYSA-N | |
Source | PubChem | |
URL | https://pubchem.ncbi.nlm.nih.gov | |
Description | Data deposited in or computed by PubChem | |
Canonical SMILES |
C=CCCCCCCCCCCC(=O)O | |
Source | PubChem | |
URL | https://pubchem.ncbi.nlm.nih.gov | |
Description | Data deposited in or computed by PubChem | |
Molecular Formula |
C13H24O2 | |
Source | PubChem | |
URL | https://pubchem.ncbi.nlm.nih.gov | |
Description | Data deposited in or computed by PubChem | |
DSSTOX Substance ID |
DTXSID80415239 | |
Record name | 12-tridecenoic acid | |
Source | EPA DSSTox | |
URL | https://comptox.epa.gov/dashboard/DTXSID80415239 | |
Description | DSSTox provides a high quality public chemistry resource for supporting improved predictive toxicology. | |
Molecular Weight |
212.33 g/mol | |
Source | PubChem | |
URL | https://pubchem.ncbi.nlm.nih.gov | |
Description | Data deposited in or computed by PubChem | |
CAS No. |
6006-06-0 | |
Record name | 12-tridecenoic acid | |
Source | EPA DSSTox | |
URL | https://comptox.epa.gov/dashboard/DTXSID80415239 | |
Description | DSSTox provides a high quality public chemistry resource for supporting improved predictive toxicology. | |
Retrosynthesis Analysis
AI-Powered Synthesis Planning: Our tool employs the Template_relevance Pistachio, Template_relevance Bkms_metabolic, Template_relevance Pistachio_ringbreaker, Template_relevance Reaxys, Template_relevance Reaxys_biocatalysis model, leveraging a vast database of chemical reactions to predict feasible synthetic routes.
One-Step Synthesis Focus: Specifically designed for one-step synthesis, it provides concise and direct routes for your target compounds, streamlining the synthesis process.
Accurate Predictions: Utilizing the extensive PISTACHIO, BKMS_METABOLIC, PISTACHIO_RINGBREAKER, REAXYS, REAXYS_BIOCATALYSIS database, our tool offers high-accuracy predictions, reflecting the latest in chemical research and data.
Strategy Settings
Precursor scoring | Relevance Heuristic |
---|---|
Min. plausibility | 0.01 |
Model | Template_relevance |
Template Set | Pistachio/Bkms_metabolic/Pistachio_ringbreaker/Reaxys/Reaxys_biocatalysis |
Top-N result to add to graph | 6 |
Feasible Synthetic Routes
Q1: What makes 11-oxo-12-tridecenoic acid interesting for antitumor research?
A1: The study highlights that both 11-oxo-12-tridecenoic acid (IXa) and 10-oxo-11-dodecenoic acid (IXb) exhibit antitumor activity. [] Although the exact mechanism of action is not elucidated in this paper, the presence of the α,β-unsaturated ketone moiety within their structures could be a key factor contributing to their biological activity. This functional group is found in various natural and synthetic compounds with known anticancer properties. Further research is needed to understand the specific ways in which these compounds interact with cancer cells and the downstream effects leading to tumor suppression.
Disclaimer and Information on In-Vitro Research Products
Please be aware that all articles and product information presented on BenchChem are intended solely for informational purposes. The products available for purchase on BenchChem are specifically designed for in-vitro studies, which are conducted outside of living organisms. In-vitro studies, derived from the Latin term "in glass," involve experiments performed in controlled laboratory settings using cells or tissues. It is important to note that these products are not categorized as medicines or drugs, and they have not received approval from the FDA for the prevention, treatment, or cure of any medical condition, ailment, or disease. We must emphasize that any form of bodily introduction of these products into humans or animals is strictly prohibited by law. It is essential to adhere to these guidelines to ensure compliance with legal and ethical standards in research and experimentation.