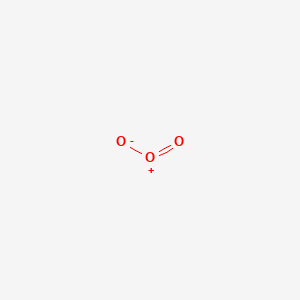
Ozone
Overview
Description
Ozone, also known as trioxygen, is a molecule composed of three oxygen atoms (O₃). It is a pale blue gas with a distinctively pungent smell. This compound is an allotrope of oxygen that is much less stable than the diatomic oxygen (O₂), breaking down in the lower atmosphere to O₂. This compound is formed from dioxygen by the action of ultraviolet light and electrical discharges within the Earth’s atmosphere. It is present in very low concentrations throughout the atmosphere, with its highest concentration in the this compound layer of the stratosphere, which absorbs most of the Sun’s ultraviolet radiation .
Preparation Methods
Synthetic Routes and Reaction Conditions: Ozone can be produced by several methods:
Electrochemical Method: This involves the decomposition of molecular oxygen into atomic oxygen using a platinum-coated lead dioxide electrode.
Ultraviolet Ray Method: Ultraviolet lamps with a specific range of wavelengths are used to decompose molecular oxygen into atomic oxygen.
Plasma Discharge Method: Secondary streamers in a plasma arc provide the necessary energy for the decomposition of molecular oxygen.
Industrial Production Methods: this compound is industrially produced using this compound generators, which typically employ the silent discharge method. This method involves passing oxygen or air through a high-voltage electrical discharge, which splits the oxygen molecules into individual atoms that then recombine to form this compound .
Types of Reactions:
Oxidation: this compound is a powerful oxidizing agent. It reacts with various organic and inorganic substances, often resulting in the formation of oxygen and other oxidation products.
Reduction: this compound can be reduced to oxygen in the presence of reducing agents.
Substitution: this compound can participate in substitution reactions, particularly with unsaturated organic compounds.
Common Reagents and Conditions:
Oxidation Reactions: this compound reacts with alkenes to form ozonides, which can be further decomposed to carbonyl compounds in the presence of reducing agents like zinc and acetic acid.
Reduction Reactions: this compound can be reduced by hydrogen peroxide to form oxygen and water.
Substitution Reactions: this compound reacts with unsaturated hydrocarbons under mild conditions to form ozonides.
Major Products:
Oxidation: Carbonyl compounds, carboxylic acids, and ketones.
Reduction: Oxygen and water.
Substitution: Ozonides and other oxygenated products.
Scientific Research Applications
Ozone in Environmental Management
Water Treatment
This compound is widely recognized for its ability to purify water. Its application in water treatment includes:
- Disinfection : this compound effectively kills bacteria, viruses, and protozoa, making it a powerful agent for ensuring safe drinking water. Studies show that ozonation can reduce microbial load significantly, often outperforming traditional chlorination methods .
- Wastewater Treatment : this compound is used to treat wastewater by breaking down organic pollutants and disinfecting effluents before they are released into the environment. This application is particularly crucial in regions facing water scarcity and pollution .
Air Purification
This compound is also employed in air treatment systems to remove odors and harmful pollutants. Its oxidative properties allow it to neutralize volatile organic compounds (VOCs) and other airborne contaminants .
Industrial Applications of this compound
Food Industry
In the food sector, this compound serves multiple purposes:
- Decontamination : this compound is used to sanitize food processing equipment and surfaces, effectively reducing microbial contamination on fruits, vegetables, and meats .
- Preservation : Ozonated water can extend the shelf life of food products by inhibiting the growth of spoilage organisms and pathogens .
Textile and Pulp Industries
This compound is utilized in bleaching processes within the textile industry, providing an environmentally friendly alternative to chlorine-based bleaches. This method not only reduces chemical waste but also improves the quality of the final product .
Medical Applications
This compound therapy has emerged as a novel approach in integrative medicine. Its applications include:
- Wound Healing : this compound has been shown to enhance wound healing by promoting oxygenation and reducing infection rates. It facilitates the cleansing of wounds and accelerates tissue repair through its antimicrobial properties .
- Therapeutic Uses : this compound therapy is being explored for treating various conditions such as arthritis, infections, and chronic pain. Clinical studies indicate that this compound can act as an immunomodulator and enhance metabolic processes within tissues .
Table: Summary of this compound Applications
Application Area | Specific Use | Benefits |
---|---|---|
Water Treatment | Disinfection of drinking water | Effective microbial reduction |
Wastewater Treatment | Treatment of industrial effluents | Degradation of organic pollutants |
Food Industry | Sanitization of surfaces | Reduction of microbial load |
Textile Industry | Bleaching fabrics | Environmentally friendly alternative |
Medical Applications | Wound healing and infection control | Enhanced healing rates |
Mechanism of Action
Ozone exerts its effects through the formation of reactive oxygen species (ROS) and lipid ozonization products (LOPs). These molecules act as signal transducers, triggering various cellular pathways such as the Nrf2/Keap1/ARE system, the AMPK/FOXO/mTOR/Sir1 pathway, and the Nrf2/NF-kB cross talk. These pathways ultimately activate survival mechanisms at the cellular level, including antioxidant responses and anti-inflammatory effects .
Comparison with Similar Compounds
Dioxygen (O₂): The diatomic form of oxygen, which is more stable than ozone.
Sulfur Dioxide (SO₂): A compound with similar oxidizing properties but different chemical behavior.
Nitrogen Dioxide (NO₂): Another oxidizing agent with distinct reactivity compared to this compound.
Uniqueness of this compound: this compound is unique due to its strong oxidizing properties and its ability to form reactive oxygen species. Unlike dioxygen, this compound is less stable and more reactive, making it highly effective in oxidation reactions and disinfection processes .
Biological Activity
Ozone (O₃) is a triatomic molecule and a powerful oxidizing agent, recognized for its dual role as both a pollutant and a therapeutic agent. Its biological activity has garnered significant attention in recent years, particularly in the context of this compound therapy, where it is employed for various medical applications. This article delves into the biological effects of this compound, exploring its mechanisms of action, therapeutic benefits, and potential health risks.
Oxidative Stress and Signaling
This compound exerts its biological effects primarily through oxidative mechanisms. When this compound interacts with biological tissues, it can lead to the generation of reactive oxygen species (ROS) and lipid oxidation products (LOPs). These compounds serve as signaling molecules that activate various cellular pathways:
- Direct Oxidation : this compound can directly oxidize polyunsaturated fatty acids, proteins, and carbohydrates, leading to cellular responses that may enhance antioxidant defenses .
- Induction of Antioxidant Enzymes : The transient oxidative stress induced by this compound therapy stimulates the activation of nuclear erythroid factor 2 (Nrf2), which regulates the expression of antioxidant enzymes such as superoxide dismutase (SOD) and glutathione peroxidase (GPx) .
Immune Modulation
This compound therapy has been shown to modulate immune responses by enhancing the production of cytokines and activating immune cells:
- Cytokine Production : this compound increases levels of interferon-γ (IFN-γ) and interleukin-2 (IL-2), which are crucial for immune activation .
- Phagocytosis Enhancement : this compound promotes phagocytosis in neutrophils and enhances the synthesis of pro-inflammatory cytokines, thereby stimulating both innate and adaptive immune responses .
Medical Uses
This compound therapy has been explored for various medical conditions due to its bactericidal, virucidal, and fungicidal properties. Notable applications include:
- Wound Healing : this compound can enhance oxygen delivery to tissues, improving healing rates in chronic wounds.
- Pain Management : Its anti-inflammatory properties make it beneficial for conditions such as arthritis.
- Vascular Health : this compound therapy has been shown to improve blood flow by altering the rheological properties of blood, enhancing red blood cell flexibility .
Case Studies
Several clinical studies have reported positive outcomes from this compound therapy:
- Chronic Pain Management : A study involving patients with chronic lower back pain demonstrated significant pain reduction following this compound injections into the affected area.
- Diabetic Ulcers : In a clinical trial involving diabetic patients with non-healing ulcers, this compound therapy resulted in accelerated healing compared to standard treatments.
Health Risks and Environmental Impact
While this compound has therapeutic benefits, its exposure at high concentrations poses significant health risks:
- Respiratory Effects : Studies indicate that exposure to ambient this compound concentrations between 160 - 360 µg/m³ can lead to reduced pulmonary function parameters . For instance, an 8-hour exposure at 120 µg/m³ may induce a 5% decrement in forced expiratory volume (FEV1) among sensitive populations .
- Long-term Exposure Risks : Prolonged exposure to elevated this compound levels is linked to chronic respiratory diseases and increased hospital admissions due to respiratory distress .
Data Table: Summary of this compound’s Biological Effects
Biological Activity | Mechanism | Therapeutic Application | Health Risk |
---|---|---|---|
Antimicrobial Action | Direct oxidative damage | Wound healing | Respiratory irritation |
Immune System Activation | Cytokine release | Pain management | Decreased lung function |
Vascular Modulation | Improved blood flow | Cardiovascular health | Long-term respiratory effects |
Antioxidant Enzyme Induction | Nrf2 activation | Chronic disease management | Potential oxidative stress |
Chemical Reactions Analysis
Catalytic Ozone Depletion in the Stratosphere
Chlorine radicals drive this compound destruction through a catalytic cycle involving two key steps :
Step | Reaction | Role of Chlorine |
---|---|---|
1 | This compound decomposition | |
2 | Chlorine regeneration |
A single chlorine atom destroys ~100,000 this compound molecules by regenerating through this cycle. This process reduces UV absorption capacity, increasing surface UV radiation by 6-12% in midlatitudes .
Reactions with Human Skin Lipids
This compound reacts with squalene (C₃₀H₅₀), the dominant unsaturated skin lipid, via Criegee mechanisms :
Key Products and Concentrations (15 ppb O₃ exposure)
Product | Structure | Steady-State Mixing Ratio |
---|---|---|
6-Methyl-5-hepten-2-one (6-MHO) | 1.7 ppb | |
4-Oxopentanal (4-OPA) | 0.8 ppb | |
Hydroxyacetone | <1 ppb | |
1,4-Butanedial | 0.5 ppb |
Experiments in simulated offices showed:
-
Forehead emissions exceed cheek/forearm due to 35% higher squalene density
-
Post-occupancy 4-OPA increases (0.5→0.8 ppb) as surface-bound precursors react further
Aqueous-Phase Reaction Mechanisms
In water, this compound undergoes complex radical-driven pathways :
Primary Reaction Sequence
This initiates a chain reaction producing hydroxyl radicals (- OH), with secondary steps:
Environmental and Health Implications
-
Stratospheric : 1% this compound loss ≈ 2% UV-B increase, elevating skin cancer risks
-
Indoor air : O₃-squalene reactions reduce this compound by 15-30% but generate respiratory irritants (e.g., 4-OPA LC₅₀ = 12 ppm)
-
Water treatment : Hydroxyl radicals from aqueous this compound oxidize pollutants 10⁴× faster than molecular O₃
These interconnected reaction pathways underscore this compound’s dual role as both an atmospheric shield and a proximate health hazard when reacting with biological systems. Experimental data from chamber studies and kinetic analyses provide quantitative constraints on its reactivity across phases.
Q & A
Q. Basic: How should researchers design experiments to investigate ozone depletion mechanisms in controlled settings?
Methodological Answer:
To study this compound depletion, researchers should employ interdisciplinary approaches integrating atmospheric chemistry and radiative transfer models. Experimental designs often involve simulating stratospheric conditions (e.g., polar stratospheric cloud formation) in reaction chambers, paired with UV spectroscopy to monitor this compound degradation kinetics. For educational frameworks, structured investigations include data interpretation exercises (e.g., analyzing historical this compound concentration datasets) and constructing models to link chlorofluorocarbon (CFC) emissions to this compound layer thinning . Key steps:
- Hypothesis formulation : Link anthropogenic emissions to catalytic this compound destruction cycles.
- Data collection : Use satellite-derived this compound monitoring instruments (e.g., TOMS, OMI) for longitudinal data.
- Model validation : Compare experimental results with computational models like the Montreal Protocol’s Photochemical Model .
Q. Basic: What statistical methods are recommended for determining background tropospheric this compound concentrations in heterogeneous environments?
Methodological Answer:
Background this compound levels require rigorous statistical filtering to distinguish natural variability from anthropogenic influences. A phased approach includes:
Data preprocessing : Remove outliers (e.g., spikes from industrial plumes) and replace missing values with detection limits .
Trend analysis : Apply spline functions or moving averages to isolate long-term trends from seasonal cycles.
Correlation analysis : Identify confounding variables (e.g., NOx levels, temperature) using multivariate regression .
Phase portrait construction : Visualize this compound dynamics against key drivers (e.g., solar radiation, wind patterns) to identify stable states .
Table 1 : Key Parameters for Background this compound Analysis
Parameter | Method | Tool/Model |
---|---|---|
Outlier removal | Tukey’s fences | Python/R scripts |
Trend decomposition | Seasonal-Trend Decomposition | STL (Statsmodels) |
Correlation | Spearman’s rank correlation | SPSS/MATLAB |
Q. Advanced: How can researchers reconcile contradictory findings in meta-analyses of this compound’s health effects?
Methodological Answer:
Contradictions often arise from heterogeneity in study populations, exposure metrics, or confounding variables (e.g., PM2.5 co-exposure). A systematic protocol includes:
- PICO framework : Define Population, Intervention (this compound exposure), Comparator, and Outcomes uniformly .
- Heterogeneity quantification : Use I² statistics and Cochran’s Q test to assess variability. If I² > 50%, apply random-effects models .
- Bias adjustment : Conduct Egger’s regression to detect publication bias and adjust via trim-and-fill analysis .
- Subgroup analysis : Stratify by exposure duration (acute vs. chronic) or demographic factors (e.g., asthmatic populations) .
Q. Advanced: What data assimilation algorithms improve short-term this compound forecasting accuracy despite model uncertainties?
Methodological Answer:
Model uncertainties (e.g., emission inventories, boundary layer dynamics) limit this compound prediction reliability. Advanced assimilation techniques include:
- Ensemble Kalman Filter (EnKF) : Perturb model parameters (e.g., NOx emissions, deposition rates) to generate error covariance matrices .
- 4D-Variational (4D-Var) : Minimize cost functions over time windows, integrating satellite this compound profiles (e.g., AIRS, IASI) .
- Hybrid approaches : Combine EnKF’s parameter perturbation with 4D-Var’s gradient-based optimization for stiff chemistry-transport models .
Table 2 : Performance Metrics for Assimilation Algorithms
Algorithm | Strengths | Limitations |
---|---|---|
Optimal Interpolation | Fast computation | Ignores model dynamics |
EnKF | Handles nonlinearities | Computational expense |
4D-Var | Integrates temporal continuity | Sensitive to initial conditions |
Q. Advanced: How can Response Surface Methodology (RSM) optimize this compound-based wastewater treatment processes?
Methodological Answer:
RSM identifies optimal this compound dosing by modeling interactions between variables (e.g., pH, contact time). Steps include:
Central Composite Design (CCD) : Define experimental ranges for variables (e.g., this compound concentration: 2–10 mg/L) .
Model fitting : Use quadratic regression to relate variables to outcomes (e.g., COD removal efficiency).
Sensitivity analysis : Identify critical factors via Pareto charts .
Validation : Confirm predictions with pilot-scale reactors.
Equation :
where : this compound dose, : reaction time .
Q. Advanced: What methodologies calculate this compound critical levels for multi-species Mediterranean ecosystems?
Methodological Answer:
Critical levels (CLs) for this compound damage to vegetation integrate stomatal flux modeling and dose-response functions:
Stomatal conductance (gₛ) modeling : Use the Jarvis-Stewart algorithm parameterized with field measurements (e.g., soil moisture, VPD) .
Exposure-Response Functions : Relate cumulative this compound flux (Phytotoxic this compound Dose, POD) to biomass loss via linear regression .
CL derivation : Set thresholds (e.g., POD₀ = 4 mmol/m²) where biomass loss exceeds 5% .
Q. Basic: How should epidemiological studies address confounding factors when assessing this compound’s synergistic effects with PM2.5?
Methodological Answer:
Synergistic effects require stratified analysis and counterfactual scenarios:
- Case-crossover design : Control for time-invariant confounders (e.g., socioeconomic status) .
- Multipollutant models : Include interaction terms (e.g., O₃ × PM2.5) in Poisson regression .
- Sensitivity analysis : Test robustness by varying exposure lag periods (0–3 days) .
Q. Advanced: What experimental frameworks quantify this compound’s role in allergen sensitization?
Methodological Answer:
Controlled exposure studies in chambers pair this compound with allergens (e.g., dust mites):
Dose-response curves : Measure IgE levels and lung function (FEV1) post-exposure .
Mechanistic assays : Analyze oxidative stress markers (8-isoprostane) in bronchial lavage .
Cohort tracking : Follow at-risk populations (e.g., children) longitudinally to assess sensitization onset .
Properties
CAS No. |
10028-15-6 |
---|---|
Molecular Formula |
O3 |
Molecular Weight |
47.998 g/mol |
IUPAC Name |
trioxirane |
InChI |
InChI=1S/O3/c1-2-3-1 |
InChI Key |
XQOAKYYZMDCSIA-UHFFFAOYSA-N |
SMILES |
[O-][O+]=O |
Canonical SMILES |
O1OO1 |
boiling_point |
-169.6 °F at 760 mmHg (EPA, 1998) -111.9 °C -112 °C -169 °F |
Color/Form |
COLORLESS GAS; DARK BLUE LIQ; BLUE-BLACK CRYSTALS BLUISH GAS Colorless to blue gas. |
density |
1.614 at -319.7 °F (NTP, 1992) - Denser than water; will sink GAS: 2.144 G/L @ 0 °C; LIQ: 1.614 G/L @ -195.4 °C 1.614 at -319.7 °F 1.66(relative gas density) |
melting_point |
-251 °C |
Key on ui other cas no. |
10028-15-6 |
physical_description |
Liquid |
Pictograms |
Oxidizer; Acute Toxic; Irritant; Health Hazard; Environmental Hazard |
shelf_life |
UNSTABLE GAS ... @ NORMAL TEMP DECOMP TO BIATOMIC OXYGEN. ... IN LIQ OR SOLID PHASE PARTICULARLY UNSTABLE. ALTHOUGH STABILITY OF OZONE IN AQ SOLN DECR AS ALKALINITY RISES, THIS EFFECT IS REVERSED @ HIGH CONCN; T/2 OF OZONE IS 2 MIN IN 1 N SODIUM HYDROXIDE; IT IS INCR TO 83 HR IN 20 N SODIUM HYDROXIDE Liquefiable at -12 °C. |
solubility |
3e-05 g/100 g at 68 °F (NTP, 1992) 49 CC/100 CC WATER AT 0 °C; SOL IN ALKALINE SOLVENTS, OILS Solubility in water: none (32 °F): 0.001% |
Synonyms |
Ground Level Ozone Level Ozone, Ground Level Ozone, Low Low Level Ozone Ozone Ozone, Ground Level Ozone, Low Level Ozone, Tropospheric Tropospheric Ozone |
vapor_density |
1.7 (EPA, 1998) - Heavier than air; will sink (Relative to Air) Relative vapor density (air = 1): 1.6 1.66 |
vapor_pressure |
41257 mmHg at 10.4 °F (EPA, 1998) >1 atm |
Origin of Product |
United States |
Synthesis routes and methods I
Procedure details
Synthesis routes and methods II
Procedure details
Disclaimer and Information on In-Vitro Research Products
Please be aware that all articles and product information presented on BenchChem are intended solely for informational purposes. The products available for purchase on BenchChem are specifically designed for in-vitro studies, which are conducted outside of living organisms. In-vitro studies, derived from the Latin term "in glass," involve experiments performed in controlled laboratory settings using cells or tissues. It is important to note that these products are not categorized as medicines or drugs, and they have not received approval from the FDA for the prevention, treatment, or cure of any medical condition, ailment, or disease. We must emphasize that any form of bodily introduction of these products into humans or animals is strictly prohibited by law. It is essential to adhere to these guidelines to ensure compliance with legal and ethical standards in research and experimentation.