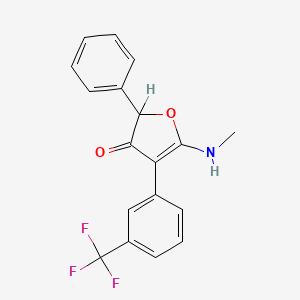
Flurtamone
Overview
Description
Flurtamone is a herbicidal active substance used primarily for controlling broad-leaved weeds and grasses. It was included in Annex I of Directive 91/414/EEC on September 25, 2003, and came into force on January 1, 2004 . This compound is known for its low aqueous solubility and low volatility, making it less likely to leach into groundwater .
Mechanism of Action
Target of Action
Flurtamone primarily targets the enzyme Phytoene Desaturase (PD) . PD is a membrane-bound enzyme in the carotenogenic pathway that catalyzes the hydrogen abstraction step at the first C40 precursor of β-carotene . This enzyme plays a crucial role in the biosynthesis of carotenoids, which are essential pigments in plants.
Mode of Action
This compound acts as a bleaching herbicide . It inhibits the activity of Phytoene Desaturase, thereby disrupting the carotenogenic pathway . This disruption prevents the synthesis of carotenoids, leading to the bleaching of the plant tissues.
Biochemical Pathways
By inhibiting Phytoene Desaturase, this compound affects the carotenogenic pathway . Carotenoids are vital for photosynthesis as they absorb light energy and protect the chlorophyll from photo-damage. When this compound inhibits the synthesis of carotenoids, it leads to the bleaching of plant tissues and eventually the death of the plant.
Pharmacokinetics
This compound has a low aqueous solubility and low volatility .
Result of Action
The primary result of this compound’s action is the control of broad-leaved weeds and grasses . By inhibiting the synthesis of carotenoids, this compound causes the bleaching of plant tissues, leading to the death of the plant. This makes it an effective herbicide for controlling unwanted vegetation.
Action Environment
This compound is used as a pre-emergence or post-emergence herbicide . It is typically applied pre-planting and soil-incorporated to control broad-leaved and some grasses . The efficacy of this compound is influenced by environmental factors such as soil type, temperature, and moisture levels.
Biochemical Analysis
Biochemical Properties
Flurtamone plays a crucial role in biochemical reactions by inhibiting the synthesis of carotenoids, which are essential pigments in plants. This inhibition disrupts the photosynthetic process, leading to the death of the plant. This compound interacts with various enzymes and proteins involved in the carotenoid biosynthesis pathway. Specifically, it inhibits the enzyme phytoene desaturase, which is responsible for the desaturation of phytoene to produce carotenoids. This interaction prevents the formation of carotenoids, leading to the accumulation of phytoene and the subsequent death of the plant .
Cellular Effects
This compound affects various types of cells and cellular processes. In plant cells, it disrupts the photosynthetic process by inhibiting carotenoid synthesis. This leads to a reduction in chlorophyll content and impairs the plant’s ability to capture light energy, ultimately causing cell death. Additionally, this compound influences cell signaling pathways by affecting the expression of genes involved in stress responses and metabolic processes. It has been observed to alter the expression of genes related to oxidative stress, leading to an increase in reactive oxygen species and subsequent cellular damage .
Molecular Mechanism
The molecular mechanism of this compound involves its binding to the enzyme phytoene desaturase, inhibiting its activity. This inhibition prevents the conversion of phytoene to carotenoids, leading to the accumulation of phytoene and the disruption of the carotenoid biosynthesis pathway. This compound’s binding to phytoene desaturase is highly specific, and it acts as a competitive inhibitor, competing with the natural substrate for the enzyme’s active site. This binding interaction is crucial for this compound’s herbicidal activity, as it effectively blocks the production of carotenoids, essential for photosynthesis and plant survival .
Temporal Effects in Laboratory Settings
In laboratory settings, the effects of this compound have been observed to change over time. The stability and degradation of this compound play a significant role in its long-term effects on cellular function. Studies have shown that this compound is relatively stable under laboratory conditions, with minimal degradation over time. Prolonged exposure to this compound can lead to the accumulation of phytoene and other intermediates in the carotenoid biosynthesis pathway, resulting in long-term cellular damage and impaired photosynthetic activity. These temporal effects highlight the importance of considering the duration of exposure when assessing the impact of this compound on cellular function .
Dosage Effects in Animal Models
The effects of this compound vary with different dosages in animal models. At low doses, this compound has minimal impact on cellular function and overall health. At higher doses, it can cause toxic effects and adverse reactions. Studies in animal models have shown that high doses of this compound can lead to liver and kidney damage, as well as alterations in metabolic processes. These dosage effects highlight the importance of determining the appropriate dosage levels to minimize potential toxic effects while maintaining the herbicidal efficacy of this compound .
Metabolic Pathways
This compound is involved in the carotenoid biosynthesis pathway, where it inhibits the enzyme phytoene desaturase. This inhibition disrupts the conversion of phytoene to carotenoids, leading to the accumulation of phytoene and the subsequent death of the plant. This compound’s interaction with phytoene desaturase is highly specific, and it acts as a competitive inhibitor, competing with the natural substrate for the enzyme’s active site. This interaction is crucial for this compound’s herbicidal activity, as it effectively blocks the production of carotenoids, essential for photosynthesis and plant survival .
Transport and Distribution
This compound is transported and distributed within cells and tissues through various mechanisms. It is absorbed by plant roots and translocated to the shoots, where it exerts its herbicidal activity. This compound interacts with transporters and binding proteins that facilitate its movement within the plant. Additionally, this compound’s localization and accumulation in specific tissues and compartments play a crucial role in its herbicidal efficacy. Studies have shown that this compound accumulates in the chloroplasts, where it inhibits carotenoid synthesis and disrupts the photosynthetic process .
Subcellular Localization
The subcellular localization of this compound is primarily in the chloroplasts, where it exerts its herbicidal activity. This compound’s targeting to the chloroplasts is facilitated by specific targeting signals and post-translational modifications that direct it to this organelle. Once in the chloroplasts, this compound inhibits the enzyme phytoene desaturase, preventing the synthesis of carotenoids and disrupting the photosynthetic process. This subcellular localization is crucial for this compound’s herbicidal activity, as it ensures that the compound reaches its target site and effectively inhibits carotenoid synthesis .
Preparation Methods
Flurtamone is synthesized through a series of chemical reactions involving specific reagents and conditions. The synthetic route typically involves the formation of a chiral molecule existing in R- and S-forms. The chemical formula for this compound is C₁₈H₁₄F₃NO₂ . Industrial production methods often involve large-scale synthesis in controlled environments to ensure the purity and efficacy of the herbicide.
Chemical Reactions Analysis
Flurtamone undergoes various chemical reactions, including:
Oxidation: this compound can be oxidized under specific conditions, leading to the formation of different metabolites.
Reduction: This compound can also undergo reduction reactions, although these are less common.
Substitution: this compound can participate in substitution reactions, where one functional group is replaced by another. Common reagents used in these reactions include oxidizing agents, reducing agents, and various catalysts.
Scientific Research Applications
Flurtamone has a wide range of applications in scientific research, including:
Chemistry: Used as a model compound to study herbicidal activity and environmental fate.
Biology: Investigated for its effects on plant physiology and weed control mechanisms.
Medicine: Limited applications, primarily in studying the toxicological effects of herbicides.
Comparison with Similar Compounds
Flurtamone is often compared with other herbicides such as Diflufenican and Metazachlor. While all these compounds are used for weed control, this compound is unique due to its specific mode of action and environmental behavior. Unlike Diflufenican, which has a broader spectrum of activity, this compound is more selective and less likely to persist in the environment .
Similar Compounds
- Diflufenican
- Metazachlor
- Isoxaflutole
This compound stands out for its low volatility and reduced risk of groundwater contamination, making it a preferred choice in certain agricultural settings .
Properties
IUPAC Name |
5-(methylamino)-2-phenyl-4-[3-(trifluoromethyl)phenyl]furan-3-one | |
---|---|---|
Source | PubChem | |
URL | https://pubchem.ncbi.nlm.nih.gov | |
Description | Data deposited in or computed by PubChem | |
InChI |
InChI=1S/C18H14F3NO2/c1-22-17-14(12-8-5-9-13(10-12)18(19,20)21)15(23)16(24-17)11-6-3-2-4-7-11/h2-10,16,22H,1H3 | |
Source | PubChem | |
URL | https://pubchem.ncbi.nlm.nih.gov | |
Description | Data deposited in or computed by PubChem | |
InChI Key |
NYRMIJKDBAQCHC-UHFFFAOYSA-N | |
Source | PubChem | |
URL | https://pubchem.ncbi.nlm.nih.gov | |
Description | Data deposited in or computed by PubChem | |
Canonical SMILES |
CNC1=C(C(=O)C(O1)C2=CC=CC=C2)C3=CC(=CC=C3)C(F)(F)F | |
Source | PubChem | |
URL | https://pubchem.ncbi.nlm.nih.gov | |
Description | Data deposited in or computed by PubChem | |
Molecular Formula |
C18H14F3NO2 | |
Source | PubChem | |
URL | https://pubchem.ncbi.nlm.nih.gov | |
Description | Data deposited in or computed by PubChem | |
DSSTOX Substance ID |
DTXSID5058228 | |
Record name | Flurtamone | |
Source | EPA DSSTox | |
URL | https://comptox.epa.gov/dashboard/DTXSID5058228 | |
Description | DSSTox provides a high quality public chemistry resource for supporting improved predictive toxicology. | |
Molecular Weight |
333.3 g/mol | |
Source | PubChem | |
URL | https://pubchem.ncbi.nlm.nih.gov | |
Description | Data deposited in or computed by PubChem | |
CAS No. |
96525-23-4 | |
Record name | Flurtamone | |
Source | CAS Common Chemistry | |
URL | https://commonchemistry.cas.org/detail?cas_rn=96525-23-4 | |
Description | CAS Common Chemistry is an open community resource for accessing chemical information. Nearly 500,000 chemical substances from CAS REGISTRY cover areas of community interest, including common and frequently regulated chemicals, and those relevant to high school and undergraduate chemistry classes. This chemical information, curated by our expert scientists, is provided in alignment with our mission as a division of the American Chemical Society. | |
Explanation | The data from CAS Common Chemistry is provided under a CC-BY-NC 4.0 license, unless otherwise stated. | |
Record name | Flurtamone [ISO:ANSI:BSI] | |
Source | ChemIDplus | |
URL | https://pubchem.ncbi.nlm.nih.gov/substance/?source=chemidplus&sourceid=0096525234 | |
Description | ChemIDplus is a free, web search system that provides access to the structure and nomenclature authority files used for the identification of chemical substances cited in National Library of Medicine (NLM) databases, including the TOXNET system. | |
Record name | Flurtamone | |
Source | EPA DSSTox | |
URL | https://comptox.epa.gov/dashboard/DTXSID5058228 | |
Description | DSSTox provides a high quality public chemistry resource for supporting improved predictive toxicology. | |
Record name | 3(2H)-Furanone, 5-(methylamino)-2-phenyl-4-[3-(trifluoromethyl)phenyl] | |
Source | European Chemicals Agency (ECHA) | |
URL | https://echa.europa.eu/substance-information/-/substanceinfo/100.117.126 | |
Description | The European Chemicals Agency (ECHA) is an agency of the European Union which is the driving force among regulatory authorities in implementing the EU's groundbreaking chemicals legislation for the benefit of human health and the environment as well as for innovation and competitiveness. | |
Explanation | Use of the information, documents and data from the ECHA website is subject to the terms and conditions of this Legal Notice, and subject to other binding limitations provided for under applicable law, the information, documents and data made available on the ECHA website may be reproduced, distributed and/or used, totally or in part, for non-commercial purposes provided that ECHA is acknowledged as the source: "Source: European Chemicals Agency, http://echa.europa.eu/". Such acknowledgement must be included in each copy of the material. ECHA permits and encourages organisations and individuals to create links to the ECHA website under the following cumulative conditions: Links can only be made to webpages that provide a link to the Legal Notice page. | |
Record name | FLURTAMONE | |
Source | FDA Global Substance Registration System (GSRS) | |
URL | https://gsrs.ncats.nih.gov/ginas/app/beta/substances/U2379SGQ8L | |
Description | The FDA Global Substance Registration System (GSRS) enables the efficient and accurate exchange of information on what substances are in regulated products. Instead of relying on names, which vary across regulatory domains, countries, and regions, the GSRS knowledge base makes it possible for substances to be defined by standardized, scientific descriptions. | |
Explanation | Unless otherwise noted, the contents of the FDA website (www.fda.gov), both text and graphics, are not copyrighted. They are in the public domain and may be republished, reprinted and otherwise used freely by anyone without the need to obtain permission from FDA. Credit to the U.S. Food and Drug Administration as the source is appreciated but not required. | |
Synthesis routes and methods I
Procedure details
Synthesis routes and methods II
Procedure details
Synthesis routes and methods III
Procedure details
Synthesis routes and methods IV
Procedure details
Synthesis routes and methods V
Procedure details
Retrosynthesis Analysis
AI-Powered Synthesis Planning: Our tool employs the Template_relevance Pistachio, Template_relevance Bkms_metabolic, Template_relevance Pistachio_ringbreaker, Template_relevance Reaxys, Template_relevance Reaxys_biocatalysis model, leveraging a vast database of chemical reactions to predict feasible synthetic routes.
One-Step Synthesis Focus: Specifically designed for one-step synthesis, it provides concise and direct routes for your target compounds, streamlining the synthesis process.
Accurate Predictions: Utilizing the extensive PISTACHIO, BKMS_METABOLIC, PISTACHIO_RINGBREAKER, REAXYS, REAXYS_BIOCATALYSIS database, our tool offers high-accuracy predictions, reflecting the latest in chemical research and data.
Strategy Settings
Precursor scoring | Relevance Heuristic |
---|---|
Min. plausibility | 0.01 |
Model | Template_relevance |
Template Set | Pistachio/Bkms_metabolic/Pistachio_ringbreaker/Reaxys/Reaxys_biocatalysis |
Top-N result to add to graph | 6 |
Feasible Synthetic Routes
Q1: What is the primary target of Flurtamone and how does it exert its herbicidal effect?
A1: this compound targets phytoene desaturase (PDS), a key enzyme in the carotenoid biosynthesis pathway. [, , , ] By inhibiting PDS, this compound disrupts the production of colored carotenoids, leading to the accumulation of phytoene, a precursor of carotenoids. [, ] This disruption ultimately results in the bleaching of plants due to the lack of protective carotenoids, which are essential for photosynthesis. [, ]
Q2: Are there differences in the biological activity of this compound enantiomers?
A3: Yes, research has shown that the R-enantiomer of this compound exhibits significantly higher herbicidal activity compared to the S-enantiomer and the racemic mixture. [] The R-enantiomer's contribution to the overall herbicidal activity was found to be dominant, ranging from 86.3% to 97.3%. []
Q3: What is the molecular formula and weight of this compound?
A3: this compound has the molecular formula C19H16F3NO2 and a molecular weight of 347.33 g/mol.
Q4: Is there information available on the spectroscopic data of this compound?
A5: While the provided research abstracts don't detail specific spectroscopic data for this compound, techniques like NMR and mass spectrometry are commonly employed for structural elucidation and identification of this compound and its metabolites. [, , , ]
Q5: How does this compound behave under various environmental conditions?
A6: Studies have examined this compound's dissipation in different soil types, demonstrating its persistence and potential impact on the environment. [, , ] Factors like soil type, previous exposure to this compound, and microbial activity influence its degradation rate. [, ]
Q6: Are there specific formulations designed to enhance this compound's stability or efficacy?
A7: Researchers have explored clay-surfactant systems for creating slow-release formulations of this compound. [] These formulations aim to improve the herbicide's bioactivity and control its release, potentially enhancing its efficacy and reducing environmental impact. []
Q7: Does this compound possess any catalytic properties?
A7: this compound is primarily recognized for its herbicidal activity, which stems from its inhibitory action on PDS. The provided research doesn't suggest any catalytic properties associated with this compound.
Q8: Have computational methods been employed to study this compound?
A9: Yes, molecular docking studies have provided insights into this compound's interaction with PDS. [] These studies highlighted the role of specific amino acid residues in the binding of this compound enantiomers to the enzyme's active site. []
Q9: What do quantitative structure-activity relationship (QSAR) studies reveal about this compound?
A10: QSAR analyses, utilizing a set of this compound derivatives, identified lipophilicity (π) and a specific steric parameter (B2) of substituents as key factors influencing the inhibitory activity against PDS. [] This information aids in understanding how structural modifications impact this compound's potency.
Q10: How do structural modifications affect this compound's activity?
A11: Research on this compound analogs revealed that meta-substituted 4-phenyl derivatives displayed significant inhibitory activity. [] The presence of a phenyl or para-fluorophenyl group at position 2 of the furanone ring proved advantageous. [] Additionally, varying the alkyl substituents of the 5-amino group influenced activity, with the ethyl derivative showing the highest potency. []
Q11: What is the environmental fate of this compound?
A13: this compound exhibits persistence in different soil types. [, , ] Its dissipation rate varies depending on factors like soil type, previous this compound exposure, and microbial activity. [, ] Studies have shown initial rapid degradation followed by a gradual slowdown over time. [] Understanding its degradation pathway is crucial for assessing its long-term environmental impact.
Q12: How does this compound behave in different media in terms of dissolution and solubility?
A14: While the abstracts don't provide detailed data on dissolution and solubility, research on this compound formulations using clay-surfactant systems suggests efforts to modulate its release profile. [] It can be inferred that controlling this compound's dissolution rate is essential for its efficacy and environmental fate.
Q13: Are there alternative herbicides with similar modes of action to this compound?
A15: Yes, other herbicides target the carotenoid biosynthesis pathway, albeit at different steps. Examples include norflurazon, fluridone, and diflufenican. [] These herbicides also disrupt carotenoid production, leading to bleaching in susceptible plants.
Q14: Has resistance to this compound been observed in weed species?
A16: While specific information on this compound resistance isn't detailed in the abstracts, research on related bleaching herbicides, like norflurazon, highlights the potential for resistance development in weed populations. [] Understanding the mechanisms of resistance to these herbicides is crucial for developing effective weed management strategies.
Q15: Is there cross-resistance between this compound and other herbicides?
A17: Research suggests that cross-resistance can occur between herbicides targeting the same pathway. A study using a Chlamydomonas mutant with a modified phytoene desaturase gene displayed tolerance to multiple bleaching herbicides, including fluridone, This compound, and diflufenican. [] This finding emphasizes the need for diverse herbicide application strategies to combat resistance development.
Q16: Can you elaborate on the resistance mechanisms observed against herbicides like this compound that target phytoene desaturase?
A18: One of the key resistance mechanisms against PDS-inhibiting herbicides involves mutations in the PDS enzyme itself. [] These mutations can alter the herbicide binding site, reducing its affinity and effectiveness. [] For example, a study identified a mutation in the pds gene of a Synechocystis mutant that conferred resistance to norflurazon, also exhibiting slight cross-resistance to this compound. [] This mutation modified a specific arginine residue, highlighting its importance in herbicide binding. []
Q17: What are the implications of non-target site resistance (NTSR) for herbicides like this compound?
A19: NTSR presents a significant challenge for weed management as it can confer resistance to multiple herbicides with different modes of action. [, ] While the provided research primarily focuses on target-site resistance, studies on related herbicides highlight the increasing prevalence of NTSR in weed populations, including those resistant to ACCase and ALS inhibitors. [, , ] This finding underscores the need for comprehensive resistance management strategies that encompass both target-site and NTSR mechanisms.
Disclaimer and Information on In-Vitro Research Products
Please be aware that all articles and product information presented on BenchChem are intended solely for informational purposes. The products available for purchase on BenchChem are specifically designed for in-vitro studies, which are conducted outside of living organisms. In-vitro studies, derived from the Latin term "in glass," involve experiments performed in controlled laboratory settings using cells or tissues. It is important to note that these products are not categorized as medicines or drugs, and they have not received approval from the FDA for the prevention, treatment, or cure of any medical condition, ailment, or disease. We must emphasize that any form of bodily introduction of these products into humans or animals is strictly prohibited by law. It is essential to adhere to these guidelines to ensure compliance with legal and ethical standards in research and experimentation.