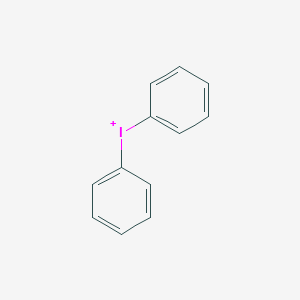
Diphenyliodonium
Overview
Description
Diphenyliodonium is a compound with the molecular formula C12H10I . It is often used in the form of its salts, such as this compound chloride . This compound is known for its role as an oxidant .
Molecular Structure Analysis
The molecular structure of this compound involves separate, nearly linear anions and cations . In the crystal of the salt, there are strong intermolecular anion-anion and anion-cation interactions .Chemical Reactions Analysis
This compound compounds are involved in various chemical reactions. For example, they have been used in the phenylation of heteroaromatic compounds . They have also been used in the reaction with free reduced flavins .Physical and Chemical Properties Analysis
This compound compounds have specific physical and chemical properties. For example, this compound chloride is a light yellow powder . It has a melting point range of 227 - 228 °C . This compound hexafluorophosphate, on the other hand, has a melting point range of 140 - 144 °C .Scientific Research Applications
1. Polymerization Initiator
Diphenyliodonium salts are utilized to initiate vigorous polymerizations, particularly in the photoinitiation of cationic cross-linking of divinyl ethers. Various systems employing this compound salts in combination with nucleophilic counter anions and zinc halides have been studied, demonstrating their significant role in direct and indirect initiating modes of polymerization (Kahveci, Tasdelen, & Yagcı, 2008).
2. Inhibition of Flavoproteins
Research has shown that compounds like this compound are potent inhibitors of flavoproteins, such as NADH-ubiquinone reductase and NADPH oxidase activity. The ability of this compound analogues to inhibit various enzymes and proteins demonstrates its potential application in studying the roles of these enzymes in cellular functions (Moulton et al., 2000).
3. Photolithography and Photoresists
This compound salts are used in photolithography, particularly as photo-acid generators (PAGs) for chemically amplified resists. The salts exhibit suitable photosensitivity and are used in environmentally friendly PAGs for 365 and 405 nm light, showcasing their applicability in the semiconductor industry and materials science (Tarumoto et al., 2005).
4. Neuroprotective and Anti-inflammatory Effects
Studies have indicated that this compound, particularly as a NADPH oxidase inhibitor, exerts potent anti-inflammatory and neuroprotective effects even at femtomolar concentrations. This is mainly through the inhibition of microglial activation and modulation of pro-inflammatory cytokine production, suggesting its potential application in neurological research and therapy (Qian et al., 2007).
5. Interaction with Flavins and Flavoproteins
This compound interacts with flavins and flavoproteins, often leading to the inhibition of various enzymes. This interaction has been explored in depth, revealing the complex dynamics between this compound, flavins, and enzyme active sites, providing insights into enzyme mechanisms and inhibition processes (Chakraborty & Massey, 2002).
6. Optical Studies and Thermochromisms
This compound salts are involved in the formation of novel hybrids with halobismuthate, leading to compounds with unique structural, optical properties, and thermochromism behaviors. This application is crucial in material science, particularly in the development of new materials with specific optical and thermal properties (Wang et al., 2018).
Mechanism of Action
The mechanism of action of diphenyliodonium compounds involves electron transfer. For instance, in the reaction with reduced flavins, the reactive flavin species was identified as the reduced anion . Another study reported the mechanism for an electron transfer photosensitization of diaryliodonium salts .
Future Directions
Research on diphenyliodonium compounds is ongoing, and these compounds have potential applications in various fields. For instance, they have been used to create photo-sensitive hybrids . They have also been used in the synthesis of new arylated bioactive heterocyclic compounds . These and other applications suggest that this compound compounds will continue to be an area of interest in future research.
Properties
IUPAC Name |
diphenyliodanium | |
---|---|---|
Source | PubChem | |
URL | https://pubchem.ncbi.nlm.nih.gov | |
Description | Data deposited in or computed by PubChem | |
InChI |
InChI=1S/C12H10I/c1-3-7-11(8-4-1)13-12-9-5-2-6-10-12/h1-10H/q+1 | |
Source | PubChem | |
URL | https://pubchem.ncbi.nlm.nih.gov | |
Description | Data deposited in or computed by PubChem | |
InChI Key |
OZLBDYMWFAHSOQ-UHFFFAOYSA-N | |
Source | PubChem | |
URL | https://pubchem.ncbi.nlm.nih.gov | |
Description | Data deposited in or computed by PubChem | |
Canonical SMILES |
C1=CC=C(C=C1)[I+]C2=CC=CC=C2 | |
Source | PubChem | |
URL | https://pubchem.ncbi.nlm.nih.gov | |
Description | Data deposited in or computed by PubChem | |
Molecular Formula |
C12H10I+ | |
Source | PubChem | |
URL | https://pubchem.ncbi.nlm.nih.gov | |
Description | Data deposited in or computed by PubChem | |
Related CAS |
1483-72-3 (chloride), 1483-73-4 (bromide), 2217-79-0 (iodide), 49723-69-5 (sulfate[1:1]), 58109-40-3 (hexafluorophosphate), 62613-15-4 (hexafluoroarsenate), 6293-66-9 (tosylate), 722-56-5 (nitrate) | |
Record name | Diphenyliodonium | |
Source | ChemIDplus | |
URL | https://pubchem.ncbi.nlm.nih.gov/substance/?source=chemidplus&sourceid=0010182840 | |
Description | ChemIDplus is a free, web search system that provides access to the structure and nomenclature authority files used for the identification of chemical substances cited in National Library of Medicine (NLM) databases, including the TOXNET system. | |
DSSTOX Substance ID |
DTXSID60144196 | |
Record name | Diphenyliodonium | |
Source | EPA DSSTox | |
URL | https://comptox.epa.gov/dashboard/DTXSID60144196 | |
Description | DSSTox provides a high quality public chemistry resource for supporting improved predictive toxicology. | |
Molecular Weight |
281.11 g/mol | |
Source | PubChem | |
URL | https://pubchem.ncbi.nlm.nih.gov | |
Description | Data deposited in or computed by PubChem | |
CAS No. |
10182-84-0 | |
Record name | Diphenyliodonium | |
Source | ChemIDplus | |
URL | https://pubchem.ncbi.nlm.nih.gov/substance/?source=chemidplus&sourceid=0010182840 | |
Description | ChemIDplus is a free, web search system that provides access to the structure and nomenclature authority files used for the identification of chemical substances cited in National Library of Medicine (NLM) databases, including the TOXNET system. | |
Record name | Diphenyliodonium | |
Source | EPA DSSTox | |
URL | https://comptox.epa.gov/dashboard/DTXSID60144196 | |
Description | DSSTox provides a high quality public chemistry resource for supporting improved predictive toxicology. | |
Synthesis routes and methods I
Procedure details
Synthesis routes and methods II
Procedure details
Retrosynthesis Analysis
AI-Powered Synthesis Planning: Our tool employs the Template_relevance Pistachio, Template_relevance Bkms_metabolic, Template_relevance Pistachio_ringbreaker, Template_relevance Reaxys, Template_relevance Reaxys_biocatalysis model, leveraging a vast database of chemical reactions to predict feasible synthetic routes.
One-Step Synthesis Focus: Specifically designed for one-step synthesis, it provides concise and direct routes for your target compounds, streamlining the synthesis process.
Accurate Predictions: Utilizing the extensive PISTACHIO, BKMS_METABOLIC, PISTACHIO_RINGBREAKER, REAXYS, REAXYS_BIOCATALYSIS database, our tool offers high-accuracy predictions, reflecting the latest in chemical research and data.
Strategy Settings
Precursor scoring | Relevance Heuristic |
---|---|
Min. plausibility | 0.01 |
Model | Template_relevance |
Template Set | Pistachio/Bkms_metabolic/Pistachio_ringbreaker/Reaxys/Reaxys_biocatalysis |
Top-N result to add to graph | 6 |
Feasible Synthetic Routes
Disclaimer and Information on In-Vitro Research Products
Please be aware that all articles and product information presented on BenchChem are intended solely for informational purposes. The products available for purchase on BenchChem are specifically designed for in-vitro studies, which are conducted outside of living organisms. In-vitro studies, derived from the Latin term "in glass," involve experiments performed in controlled laboratory settings using cells or tissues. It is important to note that these products are not categorized as medicines or drugs, and they have not received approval from the FDA for the prevention, treatment, or cure of any medical condition, ailment, or disease. We must emphasize that any form of bodily introduction of these products into humans or animals is strictly prohibited by law. It is essential to adhere to these guidelines to ensure compliance with legal and ethical standards in research and experimentation.