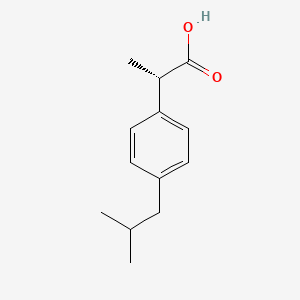
Dexibuprofen
Overview
Description
Dexibuprofen is a nonsteroidal anti-inflammatory drug (NSAID) and is the active dextrorotatory enantiomer of ibuprofen . It is used to relieve pain in conditions like Osteoarthritis, Rheumatoid Arthritis, and also to treat mild to moderate fever and pain .
Synthesis Analysis
This compound is known to be more potent than its R - (−) form and exhibits many advantages over the racemic mixture of IBU such as lower toxicity, greater clinical efficacy, and lesser variability in therapeutic effects . During the last decade, many attempts have been made to design novel formulations of DXI aimed at increasing its therapeutic benefits and minimizing the adverse effects .Molecular Structure Analysis
This compound is the active S (+)-enantiomer of ibuprofen and the only pharmacologically active enantiomer of racemic ibuprofen . The pure crystalline this compound is obtained from the racemic mixture through the innovative manufacturing process of differential crystallisation .Chemical Reactions Analysis
Like common NSAIDs, this compound is an active enantiomer of ibuprofen that suppresses the prostanoid synthesis in the inflammatory cells via inhibition of the COX-2 isoform of the arachidonic acid COX .Physical and Chemical Properties Analysis
This compound differs from the racemate in several of its physicochemical properties and has been classified as a new chemical entity with a separate ATC code . It has a slower dissolution rate in the simulated gastric and enteric juices compared with the racemic ibuprofen and displays improved oral bioavailability .Scientific Research Applications
Pharmacological Properties and Therapeutic Efficacy
Pharmacological Properties of NSAIDs : NSAIDs like ibuprofen, of which Dexibuprofen is an enantiomer, are known for their anti-inflammatory, analgesic, and antipyretic properties. These effects are primarily due to the inhibition of cyclooxygenase enzymes (COX-1 and COX-2), which play a crucial role in the synthesis of prostaglandins involved in inflammation, pain, and fever mechanisms (Moore, Pollack, & Butkerait, 2015).
Therapeutic Efficacy in Rheumatic Disorders : Ibuprofen has demonstrated significant therapeutic efficacy in treating rheumatic conditions such as rheumatoid arthritis and osteoarthritis. Its effectiveness, however, is accompanied by a safety profile that necessitates consideration of dosage and patient-specific factors to minimize adverse effects (Elizabeth F. Davies & G. Avery, 2012).
Potential Applications Beyond Pain Management
Pediatric Care : Ibuprofen is notably utilized in pediatric care for managing inflammation, mild-to-moderate pain, and fever. Its efficacy and safety in children highlight the potential of NSAIDs, including this compound, in pediatric applications, with a focus on appropriate dosing to avoid adverse reactions (M. de Martino, A. Chiarugi, A. Boner, G. Montini, & G. D. de’ Angelis, 2017).
Advanced Drug Delivery Systems : Research into novel drug delivery systems, such as nanoparticle carriers or targeted release mechanisms, could offer new ways to enhance the efficacy and reduce the side effects of NSAIDs, including this compound. This area of research holds promise for improving patient outcomes in pain management and inflammatory diseases.
Mechanism of Action
Target of Action
Dexibuprofen, also known as S (+)-ibuprofen, is a non-steroidal anti-inflammatory drug (NSAID) and is the pharmacologically active enantiomer of racemic ibuprofen . The primary target of this compound is the Cyclooxygenase (COX) enzyme . This enzyme plays a crucial role in the conversion of arachidonic acid to prostaglandins, which are key mediators of inflammation and pain .
Mode of Action
This compound exerts its therapeutic effects by suppressing the synthesis of prostanoids in inflammatory cells. This is achieved through the inhibition of the COX-2 isoform of the arachidonic acid COX . By inhibiting this enzyme, this compound reduces the production of prostaglandins, thereby alleviating pain and inflammation .
Biochemical Pathways
The primary biochemical pathway affected by this compound is the arachidonic acid pathway . In this pathway, arachidonic acid is converted into prostaglandins by the COX enzymes . This compound, by inhibiting the COX-2 isoform, reduces the production of prostaglandins . These prostaglandins (PGD2, PGE2, PGF2α, PGI2, and TXA2) are involved in various physiological processes, including inflammation and pain sensation .
Pharmacokinetics
This compound is proposed to be more pharmacologically active and tolerable with a better safety profile than ibuprofen due to a higher concentration of the active S enantiomer . It has a slower dissolution rate in simulated gastric and enteric juices compared with racemic ibuprofen, which results in improved oral bioavailability .
Result of Action
The molecular and cellular effects of this compound’s action primarily involve the reduction of inflammation and pain. By inhibiting the COX-2 isoform and subsequently reducing the production of prostaglandins, this compound alleviates symptoms of inflammation such as pain, swelling, and fever .
Action Environment
The action, efficacy, and stability of this compound can be influenced by various environmental factors. For instance, the pH of the stomach can affect the dissolution rate of this compound, which in turn impacts its bioavailability . Furthermore, factors such as diet, age, and overall health status of the individual can also influence the pharmacokinetics and pharmacodynamics of this compound.
Safety and Hazards
In the last 5 years, 4836 patients have been exposed to dexibuprofen in clinical trials and PMS trials. Only in 3.7% of patients adverse drug reactions have been reported and 3 serious adverse drug reactions (0.06%) were observed . It is proposed to be more pharmacologically active and tolerable with a better safety profile than ibuprofen due to higher concentration of active S enantiomer .
Future Directions
Dexibuprofen’s potential has been recently advocated to reduce cancer development and prevent the development of neurodegenerative diseases in addition to its anti-inflammatory properties . Many attempts have been made to design novel formulations of DXI aimed at increasing its therapeutic benefits and minimizing the adverse effects .
Biochemical Analysis
Biochemical Properties
Dexibuprofen works by blocking the effect of natural substances in the body, called cyclo-oxygenase (COX) enzymes . These enzymes help to make other chemicals in the body, called prostaglandins. Some prostaglandins are produced at sites of injury or damage, causing pain and inflammation .
Cellular Effects
This compound has significant effects on various types of cells and cellular processes. It influences cell function by impacting cell signaling pathways, gene expression, and cellular metabolism . It is known to suppress the prostanoid synthesis in inflammatory cells via inhibition of the COX-2 isoform of the arachidonic acid COX .
Molecular Mechanism
The mechanism of action of this compound involves the inhibition of COX enzymes, which are responsible for the production of prostaglandins . By blocking the effect of COX enzymes, fewer prostaglandins are produced, which means pain and inflammation are eased .
Temporal Effects in Laboratory Settings
In laboratory settings, this compound has shown to have a slower dissolution rate in simulated gastric and enteric juices compared with the racemic ibuprofen, displaying improved oral bioavailability . Over time, it has been observed to maintain its stability and effectiveness .
Dosage Effects in Animal Models
In animal models, the effects of this compound have been observed to vary with different dosages . It has been found to significantly reduce paw volume in the carrageenan-induced paw edema model, demonstrating an anti-inflammatory effect .
Metabolic Pathways
This compound is involved in the metabolic pathway of arachidonic acid, where it inhibits the COX-2 isoform, leading to a decrease in the production of prostaglandins .
Transport and Distribution
This compound is distributed throughout the body after oral administration, reaching effective concentrations in compartments where inflammation occurs . It achieves high plasma protein binding and low distribution volume .
Subcellular Localization
Given its mechanism of action, it is likely to be found in the cytoplasm where it interacts with COX enzymes to exert its effects .
Properties
IUPAC Name |
(2S)-2-[4-(2-methylpropyl)phenyl]propanoic acid | |
---|---|---|
Source | PubChem | |
URL | https://pubchem.ncbi.nlm.nih.gov | |
Description | Data deposited in or computed by PubChem | |
InChI |
InChI=1S/C13H18O2/c1-9(2)8-11-4-6-12(7-5-11)10(3)13(14)15/h4-7,9-10H,8H2,1-3H3,(H,14,15)/t10-/m0/s1 | |
Source | PubChem | |
URL | https://pubchem.ncbi.nlm.nih.gov | |
Description | Data deposited in or computed by PubChem | |
InChI Key |
HEFNNWSXXWATRW-JTQLQIEISA-N | |
Source | PubChem | |
URL | https://pubchem.ncbi.nlm.nih.gov | |
Description | Data deposited in or computed by PubChem | |
Canonical SMILES |
CC(C)CC1=CC=C(C=C1)C(C)C(=O)O | |
Source | PubChem | |
URL | https://pubchem.ncbi.nlm.nih.gov | |
Description | Data deposited in or computed by PubChem | |
Isomeric SMILES |
C[C@@H](C1=CC=C(C=C1)CC(C)C)C(=O)O | |
Source | PubChem | |
URL | https://pubchem.ncbi.nlm.nih.gov | |
Description | Data deposited in or computed by PubChem | |
Molecular Formula |
C13H18O2 | |
Source | PubChem | |
URL | https://pubchem.ncbi.nlm.nih.gov | |
Description | Data deposited in or computed by PubChem | |
DSSTOX Substance ID |
DTXSID9048724 | |
Record name | Dexibuprofen | |
Source | EPA DSSTox | |
URL | https://comptox.epa.gov/dashboard/DTXSID9048724 | |
Description | DSSTox provides a high quality public chemistry resource for supporting improved predictive toxicology. | |
Molecular Weight |
206.28 g/mol | |
Source | PubChem | |
URL | https://pubchem.ncbi.nlm.nih.gov | |
Description | Data deposited in or computed by PubChem | |
Solubility |
Insoluble | |
Record name | Dexibuprofen | |
Source | DrugBank | |
URL | https://www.drugbank.ca/drugs/DB09213 | |
Description | The DrugBank database is a unique bioinformatics and cheminformatics resource that combines detailed drug (i.e. chemical, pharmacological and pharmaceutical) data with comprehensive drug target (i.e. sequence, structure, and pathway) information. | |
Explanation | Creative Common's Attribution-NonCommercial 4.0 International License (http://creativecommons.org/licenses/by-nc/4.0/legalcode) | |
Mechanism of Action |
Like common NSAIDs, dexibuprofen is an active enantiomer of [ibuprofen] that suppresses the prostanoid synthesis in the inflammatory cells via inhibition of the COX-2 isoform of the arachidonic acid COX. For more information, refer to [ibuprofen]. | |
Record name | Dexibuprofen | |
Source | DrugBank | |
URL | https://www.drugbank.ca/drugs/DB09213 | |
Description | The DrugBank database is a unique bioinformatics and cheminformatics resource that combines detailed drug (i.e. chemical, pharmacological and pharmaceutical) data with comprehensive drug target (i.e. sequence, structure, and pathway) information. | |
Explanation | Creative Common's Attribution-NonCommercial 4.0 International License (http://creativecommons.org/licenses/by-nc/4.0/legalcode) | |
CAS No. |
51146-56-6 | |
Record name | (+)-Ibuprofen | |
Source | CAS Common Chemistry | |
URL | https://commonchemistry.cas.org/detail?cas_rn=51146-56-6 | |
Description | CAS Common Chemistry is an open community resource for accessing chemical information. Nearly 500,000 chemical substances from CAS REGISTRY cover areas of community interest, including common and frequently regulated chemicals, and those relevant to high school and undergraduate chemistry classes. This chemical information, curated by our expert scientists, is provided in alignment with our mission as a division of the American Chemical Society. | |
Explanation | The data from CAS Common Chemistry is provided under a CC-BY-NC 4.0 license, unless otherwise stated. | |
Record name | Dexibuprofen [USAN:INN:BAN] | |
Source | ChemIDplus | |
URL | https://pubchem.ncbi.nlm.nih.gov/substance/?source=chemidplus&sourceid=0051146566 | |
Description | ChemIDplus is a free, web search system that provides access to the structure and nomenclature authority files used for the identification of chemical substances cited in National Library of Medicine (NLM) databases, including the TOXNET system. | |
Record name | Dexibuprofen | |
Source | DrugBank | |
URL | https://www.drugbank.ca/drugs/DB09213 | |
Description | The DrugBank database is a unique bioinformatics and cheminformatics resource that combines detailed drug (i.e. chemical, pharmacological and pharmaceutical) data with comprehensive drug target (i.e. sequence, structure, and pathway) information. | |
Explanation | Creative Common's Attribution-NonCommercial 4.0 International License (http://creativecommons.org/licenses/by-nc/4.0/legalcode) | |
Record name | DEXIBUPROFEN | |
Source | DTP/NCI | |
URL | https://dtp.cancer.gov/dtpstandard/servlet/dwindex?searchtype=NSC&outputformat=html&searchlist=759814 | |
Description | The NCI Development Therapeutics Program (DTP) provides services and resources to the academic and private-sector research communities worldwide to facilitate the discovery and development of new cancer therapeutic agents. | |
Explanation | Unless otherwise indicated, all text within NCI products is free of copyright and may be reused without our permission. Credit the National Cancer Institute as the source. | |
Record name | Dexibuprofen | |
Source | EPA DSSTox | |
URL | https://comptox.epa.gov/dashboard/DTXSID9048724 | |
Description | DSSTox provides a high quality public chemistry resource for supporting improved predictive toxicology. | |
Record name | DEXIBUPROFEN | |
Source | FDA Global Substance Registration System (GSRS) | |
URL | https://gsrs.ncats.nih.gov/ginas/app/beta/substances/671DKG7P5S | |
Description | The FDA Global Substance Registration System (GSRS) enables the efficient and accurate exchange of information on what substances are in regulated products. Instead of relying on names, which vary across regulatory domains, countries, and regions, the GSRS knowledge base makes it possible for substances to be defined by standardized, scientific descriptions. | |
Explanation | Unless otherwise noted, the contents of the FDA website (www.fda.gov), both text and graphics, are not copyrighted. They are in the public domain and may be republished, reprinted and otherwise used freely by anyone without the need to obtain permission from FDA. Credit to the U.S. Food and Drug Administration as the source is appreciated but not required. | |
Melting Point |
49-53 | |
Record name | Dexibuprofen | |
Source | DrugBank | |
URL | https://www.drugbank.ca/drugs/DB09213 | |
Description | The DrugBank database is a unique bioinformatics and cheminformatics resource that combines detailed drug (i.e. chemical, pharmacological and pharmaceutical) data with comprehensive drug target (i.e. sequence, structure, and pathway) information. | |
Explanation | Creative Common's Attribution-NonCommercial 4.0 International License (http://creativecommons.org/licenses/by-nc/4.0/legalcode) | |
Synthesis routes and methods I
Procedure details
Synthesis routes and methods II
Procedure details
Synthesis routes and methods III
Procedure details
Synthesis routes and methods IV
Procedure details
Retrosynthesis Analysis
AI-Powered Synthesis Planning: Our tool employs the Template_relevance Pistachio, Template_relevance Bkms_metabolic, Template_relevance Pistachio_ringbreaker, Template_relevance Reaxys, Template_relevance Reaxys_biocatalysis model, leveraging a vast database of chemical reactions to predict feasible synthetic routes.
One-Step Synthesis Focus: Specifically designed for one-step synthesis, it provides concise and direct routes for your target compounds, streamlining the synthesis process.
Accurate Predictions: Utilizing the extensive PISTACHIO, BKMS_METABOLIC, PISTACHIO_RINGBREAKER, REAXYS, REAXYS_BIOCATALYSIS database, our tool offers high-accuracy predictions, reflecting the latest in chemical research and data.
Strategy Settings
Precursor scoring | Relevance Heuristic |
---|---|
Min. plausibility | 0.01 |
Model | Template_relevance |
Template Set | Pistachio/Bkms_metabolic/Pistachio_ringbreaker/Reaxys/Reaxys_biocatalysis |
Top-N result to add to graph | 6 |
Feasible Synthetic Routes
A: Dexibuprofen is a non-steroidal anti-inflammatory drug (NSAID) that exerts its therapeutic effects primarily by inhibiting cyclooxygenase (COX) enzymes. [, , ] These enzymes are responsible for the production of prostaglandins, which are lipid compounds involved in inflammation, pain, and fever. By inhibiting COX enzymes, this compound reduces prostaglandin synthesis, thereby alleviating pain and inflammation. [, , ]
A: Research suggests that this compound may also activate peroxisome proliferator-activated receptors (PPARs). [] PPAR activation can contribute to its anti-inflammatory effects by modulating the expression of genes involved in inflammatory responses. [] Additionally, studies indicate a potential role for interactions with opioid, serotonin, and nitric oxide receptors in its analgesic effects. []
ANone: this compound, also known as (S)-(+)-ibuprofen, has the molecular formula C13H18O2 and a molecular weight of 206.28 g/mol.
A: Yes, Fourier Transform Infrared Spectroscopy (FTIR) is commonly employed to characterize this compound. [, , , , ] FTIR helps identify functional groups and confirm the absence of chemical interactions in formulations. [, , ]
A: this compound, being poorly water-soluble, poses challenges in achieving desirable dissolution rates and bioavailability. [, , , ] This necessitates the exploration of compatible excipients and formulation strategies. [, , ]
A: Compatibility studies using techniques like FTIR are crucial to ensure the absence of drug-excipient interactions that could compromise stability. [, ] For instance, FTIR studies confirmed the compatibility of this compound with polymers like Eudragit E100 and TPGS in nanoparticle formulations. []
ANone: this compound itself is not known to have direct catalytic properties or applications. It primarily functions as an inhibitor of COX enzymes, which are involved in prostaglandin synthesis.
A: Molecular docking studies have been conducted to investigate the interaction of this compound and its prodrugs with COX-2, the enzyme it primarily targets. [] These simulations provide insights into binding affinities and potential for improved pharmacological activity. []
A: this compound is the S-(+)-enantiomer of Ibuprofen, and it exhibits greater potency than the racemic Ibuprofen. [, , ] This highlights the significance of chirality in influencing pharmacological activity.
A: Yes, researchers have synthesized this compound prodrugs, such as esters with antioxidant moieties, to enhance its solubility and reduce gastrointestinal side effects. [, ] These prodrugs are designed to be hydrolyzed in vivo, releasing active this compound. []
ANone: Several approaches have been explored, including:
- Solid dispersions: Combining this compound with carriers like poloxamer 407, HPMC, and SLS through spray-drying enhances solubility and dissolution. []
- Nanoparticles: Supercritical antisolvent techniques have been employed to produce this compound-Eudragit nanoparticles with improved dissolution and oral absorption. []
- Inclusion Complexes: Complexing this compound with β-cyclodextrin improves its aqueous solubility, dissolution rate, and ultimately its bioavailability. [, ]
- Emulgels: Incorporating this compound into emulgels, such as those utilizing Aloe vera gel base, offers enhanced drug loading capacity and transdermal delivery potential. []
- Hydrogel beads: Formaldehyde cross-linked hydrogel beads have shown promise as a matrix for sustained release of this compound, potentially enabling once-daily dosing. []
A: Stability studies under various conditions (temperature, humidity) are essential to assess the long-term integrity of these formulations. [, , , ] For instance, optimized this compound emulgel formulations have demonstrated good stability profiles. []
A: this compound is rapidly absorbed after oral administration, with a short half-life, necessitating frequent dosing. [, , ] It undergoes hepatic metabolism and is primarily eliminated via urine. [, ]
A: Studies reveal that co-administration with Al/Mg hydroxide antacid can decrease this compound absorption. [] Conversely, food intake has been shown to increase this compound absorption. []
A: Different routes of administration, like oral, rectal, and transdermal, can influence this compound's absorption rate and bioavailability. For instance, rectal administration via suppositories offers an alternative route, particularly in preterm infants. []
ANone: Commonly used models include:
- Carrageenan-induced paw edema in rats: This model is employed to evaluate the anti-inflammatory activity of this compound formulations, such as transdermal emulgels. []
- Acetic acid writhing test in mice: This test assesses the analgesic efficacy of this compound and its prodrugs, often comparing their potency to the parent drug. [, ]
- Hot plate latency test: This method is used to evaluate the analgesic effect of formulations like this compound-capsaicin emulgels. []
A: Numerous clinical trials have investigated the efficacy and safety of this compound in various pain conditions, including osteoarthritis, dysmenorrhea, and postoperative pain. [, , , , , , ] These trials often compare its effectiveness and tolerability to other NSAIDs, such as ibuprofen, diclofenac, and celecoxib. [, , ]
A: While generally considered safe and well-tolerated, this compound, like other NSAIDs, can cause gastrointestinal side effects in some individuals. [, , ] Its safety profile has been extensively studied in clinical trials and post-marketing surveillance. []
ANone: Research focuses on:
- Transdermal delivery: Developing emulgel formulations using permeation enhancers like menthol aims to achieve controlled and targeted delivery through the skin, potentially reducing systemic side effects. []
- Site-specific release in the gastrointestinal tract: Microparticles utilizing Eudragit L-100 have been designed to release this compound primarily in the small intestine, minimizing drug release in the stomach and potentially reducing gastrointestinal irritation. []
ANone: Currently, specific biomarkers to predict this compound efficacy or monitor treatment response are not routinely used in clinical practice.
ANone: Commonly employed techniques include:
- High-Performance Liquid Chromatography (HPLC): HPLC, often coupled with UV detection, is widely used for quantifying this compound in formulations, plasma samples, and other biological fluids. [, , , , , ]
- High-Performance Thin Layer Chromatography (HPTLC): HPTLC offers a rapid and cost-effective alternative for quantifying this compound, particularly in tablet formulations. [, ]
- Ultraviolet-Visible (UV-Vis) Spectrophotometry: UV-Vis spectrophotometry is a simple and accessible technique for determining drug content in formulations. [, ]
ANone: While specific research on the environmental impact and degradation of this compound may be limited, responsible disposal practices for pharmaceuticals are crucial to minimize potential ecological risks.
A: As a poorly water-soluble drug, this compound's dissolution rate and solubility significantly influence its bioavailability and therapeutic effectiveness. [, , , ] Formulation strategies, such as solid dispersions, nanoparticles, and inclusion complexes, aim to overcome these limitations. [, , , ]
ANone: Validation ensures the reliability and accuracy of analytical data. Key parameters include:
- Linearity: Demonstrating a linear relationship between drug concentration and instrument response over a defined range. [, , , ]
- Accuracy: Evaluating the closeness of measured values to the true value, typically assessed through recovery studies. [, , , ]
- Precision: Assessing the degree of agreement among individual test results, often determined through repeatability and intermediate precision studies. [, , , ]
- Specificity: Ensuring the method can distinguish this compound from other components in the sample matrix. [, , ]
A: Stringent quality control measures are essential throughout the development, manufacturing, and distribution of this compound formulations to ensure product consistency, safety, and efficacy. These measures adhere to established pharmaceutical guidelines and regulations. [, ]
ANone: this compound, as an NSAID, does not typically elicit significant immunogenic or allergic responses.
ANone: this compound is primarily metabolized in the liver, and its potential to induce or inhibit drug-metabolizing enzymes, such as cytochrome P450 (CYP) enzymes, should be considered when co-administered with other medications.
ANone: As a pharmaceutical compound, this compound is expected to be biocompatible within its therapeutic window. Its biodegradability is a crucial factor in assessing its environmental impact and the development of sustainable manufacturing processes.
A: Other NSAIDs, such as Ibuprofen, Naproxen, Diclofenac, and Celecoxib, serve as alternatives to this compound, each with its own pharmacological profile, efficacy, and safety considerations. [, , , ]
ANone: Proper disposal of pharmaceutical waste, including unused this compound formulations, is crucial to prevent environmental contamination and promote responsible waste management practices.
ANone: Continued research on this compound benefits from:
- Advanced analytical techniques: Development and refinement of sensitive and specific analytical methods, such as HPLC and HPTLC, to quantify this compound in various matrices. [, , , , , , , ]
- In vitro and in vivo models: Utilization of robust and clinically relevant models to study its efficacy, safety, and pharmacokinetic properties. [, , ]
- Computational tools: Employing computational chemistry and modeling approaches to gain deeper insights into its molecular interactions and guide the development of improved formulations. []
ANone: this compound's development stemmed from the recognition that it is the pharmacologically active enantiomer of racemic ibuprofen. Its introduction marked a significant advancement in pain management, offering a more potent and potentially safer alternative.
ANone: Research on this compound benefits from collaborations among:
- Pharmaceutical scientists: Focus on formulation development, analytical method development, and stability studies. [, , , , , , , , , , , , ]
- Pharmacologists: Investigate its mechanism of action, pharmacodynamics, and efficacy in preclinical models. [, , ]
- Clinicians: Conduct clinical trials to evaluate its efficacy and safety in patients with various pain conditions. [, , , , , , ]
- Computational chemists: Employ modeling and simulation techniques to understand its molecular interactions and guide drug design. []
Disclaimer and Information on In-Vitro Research Products
Please be aware that all articles and product information presented on BenchChem are intended solely for informational purposes. The products available for purchase on BenchChem are specifically designed for in-vitro studies, which are conducted outside of living organisms. In-vitro studies, derived from the Latin term "in glass," involve experiments performed in controlled laboratory settings using cells or tissues. It is important to note that these products are not categorized as medicines or drugs, and they have not received approval from the FDA for the prevention, treatment, or cure of any medical condition, ailment, or disease. We must emphasize that any form of bodily introduction of these products into humans or animals is strictly prohibited by law. It is essential to adhere to these guidelines to ensure compliance with legal and ethical standards in research and experimentation.