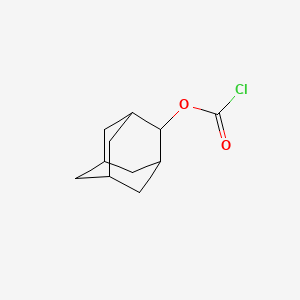
2-adamantyl Chloroformate
Overview
Description
2-adamantyl Chloroformate is an organic compound derived from adamantane, a hydrocarbon with a unique cage-like structure. This compound is characterized by the presence of a chloroformate group attached to the adamantyl moiety. It is widely used in organic synthesis due to its reactivity and stability.
Preparation Methods
Synthetic Routes and Reaction Conditions
2-adamantyl Chloroformate can be synthesized through the reaction of 2-adamantanol with phosgene. The reaction typically occurs in the presence of a base such as pyridine to neutralize the hydrochloric acid formed during the process. The general reaction is as follows:
2-adamantanol+phosgene→2-adamantyl Chloroformate+hydrochloric acid
Industrial Production Methods
Industrial production of this compound follows similar synthetic routes but on a larger scale. The process involves the careful handling of phosgene, a toxic and hazardous reagent, under controlled conditions to ensure safety and efficiency.
Chemical Reactions Analysis
Types of Reactions
2-adamantyl Chloroformate undergoes various chemical reactions, including:
Substitution Reactions: It reacts with nucleophiles such as amines and alcohols to form carbamates and carbonates, respectively.
Hydrolysis: In the presence of water, it hydrolyzes to form 2-adamantanol and carbon dioxide.
Solvolysis: It undergoes solvolysis in different solvents, leading to the formation of 2-adamantyl cation and subsequent products.
Common Reagents and Conditions
Amines: React with this compound to form carbamates.
Alcohols: React to form carbonate esters.
Water: Hydrolyzes the compound to 2-adamantanol and carbon dioxide.
Major Products Formed
Carbamates: Formed from reactions with amines.
Carbonate Esters: Formed from reactions with alcohols.
2-adamantanol: Formed from hydrolysis.
Scientific Research Applications
2-adamantyl Chloroformate has diverse applications in scientific research:
Organic Synthesis: Used as a reagent for introducing the adamantyl group into various molecules.
Medicinal Chemistry: Utilized in the synthesis of pharmaceutical intermediates and active compounds.
Material Science: Employed in the development of advanced materials with unique properties.
Catalysis: Acts as a catalyst or catalyst precursor in various chemical reactions.
Mechanism of Action
The mechanism of action of 2-adamantyl Chloroformate involves the formation of reactive intermediates such as the 2-adamantyl cation. This cation can undergo various reactions depending on the conditions and reagents present. The molecular targets and pathways involved include:
Formation of 2-adamantyl cation: Through solvolysis or reaction with nucleophiles.
Subsequent Reactions: The cation can react with nucleophiles, leading to the formation of various products.
Comparison with Similar Compounds
2-adamantyl Chloroformate can be compared with other adamantyl derivatives such as:
1-adamantyl Chloroformate: Similar in structure but with the chloroformate group attached to a different position on the adamantyl ring.
2-adamantyl Bromide: Contains a bromine atom instead of the chloroformate group.
2-adamantyl Acetate: Contains an acetate group instead of the chloroformate group.
Uniqueness
This compound is unique due to its specific reactivity and stability, making it a valuable reagent in organic synthesis and various scientific applications.
Properties
IUPAC Name |
2-adamantyl carbonochloridate | |
---|---|---|
Source | PubChem | |
URL | https://pubchem.ncbi.nlm.nih.gov | |
Description | Data deposited in or computed by PubChem | |
InChI |
InChI=1S/C11H15ClO2/c12-11(13)14-10-8-2-6-1-7(4-8)5-9(10)3-6/h6-10H,1-5H2 | |
Source | PubChem | |
URL | https://pubchem.ncbi.nlm.nih.gov | |
Description | Data deposited in or computed by PubChem | |
InChI Key |
WUVZDJSSAGWPIE-UHFFFAOYSA-N | |
Source | PubChem | |
URL | https://pubchem.ncbi.nlm.nih.gov | |
Description | Data deposited in or computed by PubChem | |
Canonical SMILES |
C1C2CC3CC1CC(C2)C3OC(=O)Cl | |
Source | PubChem | |
URL | https://pubchem.ncbi.nlm.nih.gov | |
Description | Data deposited in or computed by PubChem | |
Molecular Formula |
C11H15ClO2 | |
Source | PubChem | |
URL | https://pubchem.ncbi.nlm.nih.gov | |
Description | Data deposited in or computed by PubChem | |
DSSTOX Substance ID |
DTXSID20446845 | |
Record name | 2-adamantyl Chloroformate | |
Source | EPA DSSTox | |
URL | https://comptox.epa.gov/dashboard/DTXSID20446845 | |
Description | DSSTox provides a high quality public chemistry resource for supporting improved predictive toxicology. | |
Molecular Weight |
214.69 g/mol | |
Source | PubChem | |
URL | https://pubchem.ncbi.nlm.nih.gov | |
Description | Data deposited in or computed by PubChem | |
CAS No. |
53120-53-9 | |
Record name | 2-adamantyl Chloroformate | |
Source | EPA DSSTox | |
URL | https://comptox.epa.gov/dashboard/DTXSID20446845 | |
Description | DSSTox provides a high quality public chemistry resource for supporting improved predictive toxicology. | |
Synthesis routes and methods I
Procedure details
Synthesis routes and methods II
Procedure details
Synthesis routes and methods III
Procedure details
Synthesis routes and methods IV
Procedure details
Retrosynthesis Analysis
AI-Powered Synthesis Planning: Our tool employs the Template_relevance Pistachio, Template_relevance Bkms_metabolic, Template_relevance Pistachio_ringbreaker, Template_relevance Reaxys, Template_relevance Reaxys_biocatalysis model, leveraging a vast database of chemical reactions to predict feasible synthetic routes.
One-Step Synthesis Focus: Specifically designed for one-step synthesis, it provides concise and direct routes for your target compounds, streamlining the synthesis process.
Accurate Predictions: Utilizing the extensive PISTACHIO, BKMS_METABOLIC, PISTACHIO_RINGBREAKER, REAXYS, REAXYS_BIOCATALYSIS database, our tool offers high-accuracy predictions, reflecting the latest in chemical research and data.
Strategy Settings
Precursor scoring | Relevance Heuristic |
---|---|
Min. plausibility | 0.01 |
Model | Template_relevance |
Template Set | Pistachio/Bkms_metabolic/Pistachio_ringbreaker/Reaxys/Reaxys_biocatalysis |
Top-N result to add to graph | 6 |
Feasible Synthetic Routes
Disclaimer and Information on In-Vitro Research Products
Please be aware that all articles and product information presented on BenchChem are intended solely for informational purposes. The products available for purchase on BenchChem are specifically designed for in-vitro studies, which are conducted outside of living organisms. In-vitro studies, derived from the Latin term "in glass," involve experiments performed in controlled laboratory settings using cells or tissues. It is important to note that these products are not categorized as medicines or drugs, and they have not received approval from the FDA for the prevention, treatment, or cure of any medical condition, ailment, or disease. We must emphasize that any form of bodily introduction of these products into humans or animals is strictly prohibited by law. It is essential to adhere to these guidelines to ensure compliance with legal and ethical standards in research and experimentation.