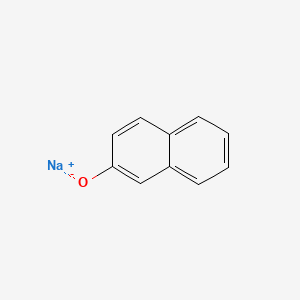
Sodium 2-naphtholate
Overview
Description
Sodium 2-naphtholate, with the chemical formula C10H7NaO, is a sodium salt of 2-naphthol. It appears as a grayish-white powder that becomes reddish or brownish upon exposure to light and air . This compound is widely used in organic synthesis and serves as a reagent in various chemical reactions .
Mechanism of Action
Target of Action
Sodium 2-naphtholate, also known as sodium naphthalen-2-olate, primarily targets naphthalene anions . These anions are selected according to their drift speed through nitrogen buffer gas in a tandem drift tube ion mobility spectrometer .
Mode of Action
The compound interacts with its targets through a process called photodetachment . This involves exposing the target anions to a pulse of tunable light that induces either photodissociation or electron photodetachment . The photodetachment action spectrum of the 2-naphtholate anion exhibits a band system spanning 380–460 nm .
Biochemical Pathways
The carboxylation reaction of sodium 2-naphthoxide is a key biochemical pathway affected by this compound . This reaction involves the formation of sodium 2-naphthoxide-CO2 complex, followed by an electrophilic attack by the carbon of the CO2 moiety on the naphthalene ring . Another pathway involves the alkylation of this compound, which yields high ratios of O/O+C alkylation when conducted in THF, in benzene, and in water .
Pharmacokinetics
It’s known that the compound is a grayish-white powder that becomes reddish or brownish on exposure to light and air . This suggests that the compound’s bioavailability may be influenced by environmental conditions such as light and air exposure.
Result of Action
The primary result of this compound’s action is the photodetachment of electrons from naphthalene anions . This process results in a series of peaks in the photodetachment action spectrum of the 2-naphtholate anion . In the carboxylation reaction, the electrophilic attack by the carbon of the CO2 moiety on the naphthalene ring leads to the formation of new compounds .
Action Environment
The action of this compound is influenced by environmental factors such as light and air exposure . For instance, the compound becomes reddish or brownish on exposure to light and air . Additionally, the alkylation of this compound yields high ratios of O/O+C alkylation when conducted in THF, in benzene, and in water , suggesting that the solvent environment can influence the compound’s action.
Preparation Methods
Synthetic Routes and Reaction Conditions: Sodium 2-naphtholate can be synthesized through the sulfonation and alkali fusion of refined naphthalene. The process involves sulfonating naphthalene with 98% sulfuric acid, followed by salting-out, alkali-fusing, acidifying, and distilling .
Industrial Production Methods: The industrial production of this compound follows a similar process, optimizing the sulfonation and alkali fusion steps to reduce production costs and minimize environmental impact .
Chemical Reactions Analysis
Types of Reactions: Sodium 2-naphtholate undergoes various chemical reactions, including:
Oxidation: It can be oxidized to form 2-naphthoquinone.
Reduction: It can be reduced to form 2-naphthol.
Substitution: It participates in nucleophilic substitution reactions, such as the Kolbe-Schmitt reaction.
Common Reagents and Conditions:
Oxidation: Common oxidizing agents include potassium permanganate and chromium trioxide.
Reduction: Common reducing agents include sodium borohydride and lithium aluminum hydride.
Substitution: The Kolbe-Schmitt reaction involves carbon dioxide and sodium hydroxide under high pressure and temperature.
Major Products:
Oxidation: 2-naphthoquinone
Reduction: 2-naphthol
Substitution: Sodium 2-hydroxy-1-naphthoate
Scientific Research Applications
Sodium 2-naphtholate has diverse applications in scientific research, including:
Chemistry: It is used as a reagent in organic synthesis and in the preparation of dyes and pigments.
Biology: It serves as a precursor for various biologically active compounds.
Medicine: It is used in the synthesis of pharmaceutical intermediates.
Industry: It is employed in the production of antioxidants, sterilants, and liquid crystal materials.
Comparison with Similar Compounds
- Sodium 1-naphtholate
- Sodium 2-naphthoxide
- Sodium β-naphtholate
Comparison: Sodium 2-naphtholate is unique due to its specific reactivity in the Kolbe-Schmitt reaction, leading to the formation of sodium 2-hydroxy-1-naphthoate. In contrast, sodium 1-naphtholate and sodium 2-naphthoxide have different reactivity patterns and are used in different synthetic applications .
Properties
CAS No. |
875-83-2 |
---|---|
Molecular Formula |
C10H8NaO |
Molecular Weight |
167.16 g/mol |
IUPAC Name |
sodium;naphthalen-2-olate |
InChI |
InChI=1S/C10H8O.Na/c11-10-6-5-8-3-1-2-4-9(8)7-10;/h1-7,11H; |
InChI Key |
ZNYPFGRLOVXIFH-UHFFFAOYSA-N |
SMILES |
C1=CC=C2C=C(C=CC2=C1)[O-].[Na+] |
Canonical SMILES |
C1=CC=C2C=C(C=CC2=C1)O.[Na] |
875-83-2 | |
Related CAS |
135-19-3 (Parent) |
Origin of Product |
United States |
Synthesis routes and methods I
Procedure details
Synthesis routes and methods II
Procedure details
Synthesis routes and methods III
Procedure details
Retrosynthesis Analysis
AI-Powered Synthesis Planning: Our tool employs the Template_relevance Pistachio, Template_relevance Bkms_metabolic, Template_relevance Pistachio_ringbreaker, Template_relevance Reaxys, Template_relevance Reaxys_biocatalysis model, leveraging a vast database of chemical reactions to predict feasible synthetic routes.
One-Step Synthesis Focus: Specifically designed for one-step synthesis, it provides concise and direct routes for your target compounds, streamlining the synthesis process.
Accurate Predictions: Utilizing the extensive PISTACHIO, BKMS_METABOLIC, PISTACHIO_RINGBREAKER, REAXYS, REAXYS_BIOCATALYSIS database, our tool offers high-accuracy predictions, reflecting the latest in chemical research and data.
Strategy Settings
Precursor scoring | Relevance Heuristic |
---|---|
Min. plausibility | 0.01 |
Model | Template_relevance |
Template Set | Pistachio/Bkms_metabolic/Pistachio_ringbreaker/Reaxys/Reaxys_biocatalysis |
Top-N result to add to graph | 6 |
Feasible Synthetic Routes
Q1: What makes sodium 2-naphtholate useful in organic synthesis?
A: this compound is the sodium salt of 2-naphthol, acting as a potent nucleophile in organic reactions. This reactivity stems from the electron-donating nature of the naphthoxide anion, making it particularly useful for alkylation and carboxylation reactions. [, , ]
Q2: Can you elaborate on the impact of macrocyclic polyethers on the alkylation of this compound?
A: Research has shown that macrocyclic polyethers, such as benzo-18-crown-6 and 4,7,13,16,21,24-hexaoxabicyclo[8.8.8]hexacosane, significantly enhance the ratio of O-alkylation to C-alkylation in reactions with this compound. [, ] This effect is attributed to the specific complexation of the sodium cation by the polyether, which facilitates the dissociation of the unreactive ion-pair aggregates and promotes the formation of the more reactive "naked" anion. []
Q3: What is the interaction between this compound and cetylpyridinium chloride in an aqueous solution?
A: Studies reveal that this compound forms a non-fluorescent complex with cetylpyridinium chloride in aqueous solutions. [] This interaction leads to a decrease in the fluorescence intensity of 2-naphtholate, especially at concentrations below the critical micelle concentration of cetylpyridinium chloride. [] At higher concentrations, dynamic interactions between 2-naphtholate ions and cetylpyridinium micelles further contribute to fluorescence quenching. []
Q4: Are there examples of this compound being employed in catalytic applications?
A: Yes, a mixture of 2-naphthol and this compound has proven effective as a bifunctional catalyst in direct aldol reactions. [] This combination facilitates the formation of hydroxyketones in high yields, highlighting the potential of this compound in catalytic organic transformations. []
Disclaimer and Information on In-Vitro Research Products
Please be aware that all articles and product information presented on BenchChem are intended solely for informational purposes. The products available for purchase on BenchChem are specifically designed for in-vitro studies, which are conducted outside of living organisms. In-vitro studies, derived from the Latin term "in glass," involve experiments performed in controlled laboratory settings using cells or tissues. It is important to note that these products are not categorized as medicines or drugs, and they have not received approval from the FDA for the prevention, treatment, or cure of any medical condition, ailment, or disease. We must emphasize that any form of bodily introduction of these products into humans or animals is strictly prohibited by law. It is essential to adhere to these guidelines to ensure compliance with legal and ethical standards in research and experimentation.