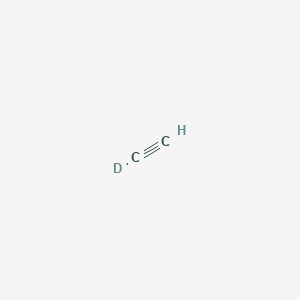
Acetylene-d1
Overview
Description
Acetylene-d1, also known as deuterated acetylene, is a compound with the molecular formula C2HD. It is a deuterium-labeled version of acetylene (C2H2), where one of the hydrogen atoms is replaced by deuterium, an isotope of hydrogen. This compound is used in various scientific research applications due to its unique properties and isotopic labeling.
Preparation Methods
Synthetic Routes and Reaction Conditions
Acetylene-d1 can be synthesized through several methods. One common method involves the reaction of calcium carbide (CaC2) with deuterium oxide (D2O). The reaction proceeds as follows: [ \text{CaC}_2 + 2\text{D}_2\text{O} \rightarrow \text{C}_2\text{D}_2 + \text{Ca(OD)}_2 ] This reaction produces deuterated acetylene (C2D2), which can then be partially deuterated to obtain this compound (C2HD).
Industrial Production Methods
In industrial settings, this compound can be produced using similar methods as those used for regular acetylene, but with deuterium-containing reagents. The production involves the use of deuterium gas (D2) in place of hydrogen gas (H2) in the synthesis process. This ensures the incorporation of deuterium into the acetylene molecule.
Chemical Reactions Analysis
Types of Reactions
Acetylene-d1 undergoes various chemical reactions, including:
Oxidation: this compound can be oxidized to form deuterated acetic acid (CD3COOD) or other deuterated organic compounds.
Reduction: It can be reduced to form deuterated ethylene (C2H4D) or deuterated ethane (C2H6D).
Substitution: this compound can participate in substitution reactions where the deuterium atom is replaced by other functional groups.
Common Reagents and Conditions
Oxidation: Common oxidizing agents include potassium permanganate (KMnO4) and ozone (O3).
Reduction: Catalysts such as palladium on carbon (Pd/C) and hydrogen gas (H2) are used for reduction reactions.
Substitution: Halogenation reagents like bromine (Br2) or chlorine (Cl2) can be used for substitution reactions.
Major Products
Oxidation: Deuterated acetic acid (CD3COOD)
Reduction: Deuterated ethylene (C2H4D) and deuterated ethane (C2H6D)
Substitution: Deuterated halogenated compounds (e.g., C2HBrD)
Scientific Research Applications
Acetylene-d1 is widely used in scientific research due to its isotopic labeling, which allows for the study of reaction mechanisms and pathways. Some applications include:
Chemistry: Used as a tracer in reaction mechanism studies to understand the behavior of acetylene in various chemical reactions.
Biology: Employed in metabolic studies to trace the incorporation of acetylene into biological molecules.
Medicine: Utilized in the development of deuterated drugs, which can have improved pharmacokinetic properties.
Industry: Used in the production of deuterated solvents and other deuterium-labeled compounds for various industrial applications.
Mechanism of Action
The mechanism of action of acetylene-d1 involves its participation in chemical reactions where the deuterium atom can influence the reaction rate and pathway. The presence of deuterium, which is heavier than hydrogen, can lead to kinetic isotope effects, where the reaction rate is altered due to the difference in bond strength and vibrational frequencies between C-D and C-H bonds. This allows researchers to study the detailed mechanisms of reactions involving acetylene.
Comparison with Similar Compounds
Similar Compounds
Acetylene (C2H2): The non-deuterated version of acetylene.
Deuterated Ethylene (C2H4D): A deuterium-labeled version of ethylene.
Deuterated Ethane (C2H6D): A deuterium-labeled version of ethane.
Uniqueness
Acetylene-d1 is unique due to its isotopic labeling, which provides distinct advantages in research applications. The presence of deuterium allows for the study of kinetic isotope effects and detailed reaction mechanisms that are not possible with non-deuterated compounds. This makes this compound a valuable tool in various scientific fields.
Properties
IUPAC Name |
deuterioethyne | |
---|---|---|
Source | PubChem | |
URL | https://pubchem.ncbi.nlm.nih.gov | |
Description | Data deposited in or computed by PubChem | |
InChI |
InChI=1S/C2H2/c1-2/h1-2H/i1D | |
Source | PubChem | |
URL | https://pubchem.ncbi.nlm.nih.gov | |
Description | Data deposited in or computed by PubChem | |
InChI Key |
HSFWRNGVRCDJHI-MICDWDOJSA-N | |
Source | PubChem | |
URL | https://pubchem.ncbi.nlm.nih.gov | |
Description | Data deposited in or computed by PubChem | |
Canonical SMILES |
C#C | |
Source | PubChem | |
URL | https://pubchem.ncbi.nlm.nih.gov | |
Description | Data deposited in or computed by PubChem | |
Isomeric SMILES |
[2H]C#C | |
Source | PubChem | |
URL | https://pubchem.ncbi.nlm.nih.gov | |
Description | Data deposited in or computed by PubChem | |
Molecular Formula |
C2H2 | |
Source | PubChem | |
URL | https://pubchem.ncbi.nlm.nih.gov | |
Description | Data deposited in or computed by PubChem | |
DSSTOX Substance ID |
DTXSID20176610 | |
Record name | Acetylene-d1 | |
Source | EPA DSSTox | |
URL | https://comptox.epa.gov/dashboard/DTXSID20176610 | |
Description | DSSTox provides a high quality public chemistry resource for supporting improved predictive toxicology. | |
Molecular Weight |
27.04 g/mol | |
Source | PubChem | |
URL | https://pubchem.ncbi.nlm.nih.gov | |
Description | Data deposited in or computed by PubChem | |
Physical Description |
Colorless gas; May have odor like garlic; [C/D/N Isotopes MSDS] | |
Record name | Acetylene-d1 | |
Source | Haz-Map, Information on Hazardous Chemicals and Occupational Diseases | |
URL | https://haz-map.com/Agents/13647 | |
Description | Haz-Map® is an occupational health database designed for health and safety professionals and for consumers seeking information about the adverse effects of workplace exposures to chemical and biological agents. | |
Explanation | Copyright (c) 2022 Haz-Map(R). All rights reserved. Unless otherwise indicated, all materials from Haz-Map are copyrighted by Haz-Map(R). No part of these materials, either text or image may be used for any purpose other than for personal use. Therefore, reproduction, modification, storage in a retrieval system or retransmission, in any form or by any means, electronic, mechanical or otherwise, for reasons other than personal use, is strictly prohibited without prior written permission. | |
CAS No. |
2210-34-6 | |
Record name | Ethyne-d | |
Source | CAS Common Chemistry | |
URL | https://commonchemistry.cas.org/detail?cas_rn=2210-34-6 | |
Description | CAS Common Chemistry is an open community resource for accessing chemical information. Nearly 500,000 chemical substances from CAS REGISTRY cover areas of community interest, including common and frequently regulated chemicals, and those relevant to high school and undergraduate chemistry classes. This chemical information, curated by our expert scientists, is provided in alignment with our mission as a division of the American Chemical Society. | |
Explanation | The data from CAS Common Chemistry is provided under a CC-BY-NC 4.0 license, unless otherwise stated. | |
Record name | Acetylene-d1 | |
Source | ChemIDplus | |
URL | https://pubchem.ncbi.nlm.nih.gov/substance/?source=chemidplus&sourceid=0002210346 | |
Description | ChemIDplus is a free, web search system that provides access to the structure and nomenclature authority files used for the identification of chemical substances cited in National Library of Medicine (NLM) databases, including the TOXNET system. | |
Record name | Acetylene-d1 | |
Source | EPA DSSTox | |
URL | https://comptox.epa.gov/dashboard/DTXSID20176610 | |
Description | DSSTox provides a high quality public chemistry resource for supporting improved predictive toxicology. | |
Synthesis routes and methods I
Procedure details
Synthesis routes and methods II
Procedure details
Synthesis routes and methods III
Procedure details
Synthesis routes and methods IV
Procedure details
Retrosynthesis Analysis
AI-Powered Synthesis Planning: Our tool employs the Template_relevance Pistachio, Template_relevance Bkms_metabolic, Template_relevance Pistachio_ringbreaker, Template_relevance Reaxys, Template_relevance Reaxys_biocatalysis model, leveraging a vast database of chemical reactions to predict feasible synthetic routes.
One-Step Synthesis Focus: Specifically designed for one-step synthesis, it provides concise and direct routes for your target compounds, streamlining the synthesis process.
Accurate Predictions: Utilizing the extensive PISTACHIO, BKMS_METABOLIC, PISTACHIO_RINGBREAKER, REAXYS, REAXYS_BIOCATALYSIS database, our tool offers high-accuracy predictions, reflecting the latest in chemical research and data.
Strategy Settings
Precursor scoring | Relevance Heuristic |
---|---|
Min. plausibility | 0.01 |
Model | Template_relevance |
Template Set | Pistachio/Bkms_metabolic/Pistachio_ringbreaker/Reaxys/Reaxys_biocatalysis |
Top-N result to add to graph | 6 |
Feasible Synthetic Routes
Q1: What is the significance of using deuterated Acetylene (C₂H₂) in studying nitrogenase activity?
A1: Deuterated Acetylene, specifically Acetylene-d2 (C₂D₂), serves as a valuable tool in nitrogenase research. Nitrogenase, the enzyme responsible for biological nitrogen fixation, can also reduce Acetylene to Ethylene. By using C₂D₂, researchers can distinguish between Ethylene produced by nitrogenase and Ethylene from other sources, such as plant biosynthesis []. This is because nitrogenase primarily converts C₂D₂ to cis-1,2-Dideuteroethylene, which can be differentiated from non-deuterated Ethylene through Fourier transform infrared spectroscopy [].
Q2: What is the structural difference between Acetylene (C₂H₂) and Acetylene-d1 (C₂HD)?
A2: this compound (C₂HD) differs from Acetylene (C₂H₂) by the substitution of one hydrogen atom with a deuterium atom. This isotopic substitution alters the molecule's vibrational frequencies, leading to distinct spectroscopic properties.
Q3: How does the presence of this compound affect the product distribution in reactions involving ethynyl radicals?
A3: Studies have shown that when Acetylene-d2 (C₂D₂) is introduced into a system containing ethynyl radicals (C₂H.), it participates in reactions leading to the formation of both this compound (C₂HD) and butadiyne-d1 (C₄HD) []. This finding highlights the occurrence of both hydrogen abstraction and displacement reactions by ethynyl radicals in the presence of acetylenic species.
Q4: Are there specific spectroscopic techniques that are particularly useful for studying this compound?
A4: Yes, several spectroscopic techniques are well-suited for studying this compound:
- Infrared Spectroscopy: Provides information on molecular vibrations and is useful for identifying and quantifying C₂HD based on its unique absorption bands [, ].
- Raman Spectroscopy: Complementary to infrared spectroscopy, offering insights into molecular vibrations and structural characteristics [, ].
- Microwave Spectroscopy: Allows for the precise determination of molecular rotational constants and structural parameters [].
Q5: How does the polymerization temperature influence the structure of Polyacetylene synthesized using a Ti(OC₄H₉)₄–Al(C₂H₅)₃ catalyst system?
A5: Infrared spectral analysis of Polyacetylene, Poly(acetylene-d2), and copolymers synthesized using a Ti(OC₄H₉)₄–Al(C₂H₅)₃ catalyst system suggests a temperature-dependent structural variation []. Polymers produced at lower temperatures (below -78°C) exhibit spectral features consistent with either an all cis-transoid or all trans-cisoid configuration. In contrast, polymers synthesized at higher temperatures (above 150°C) display spectra indicative of an all-trans structure [].
Q6: What insights into acetylene pyrolysis have been gained through shock tube studies coupled with time-of-flight mass spectrometry?
A6: Shock tube experiments, coupled with time-of-flight mass spectrometry, have revealed that Acetylene pyrolysis proceeds through a sequential formation of higher polyynes []. The proposed pathway involves the initial formation of C₄H₃, followed by its conversion to C₄H₂, and subsequent formation of C₆H₂, C₈H₂, and so on []. This study highlighted the formation of C₄H₃ as a key intermediate in Acetylene pyrolysis.
Q7: What is the role of hydrogen atoms in the thermal decomposition of Acetylene?
A7: Hydrogen atoms play a crucial role in the thermal decomposition of Acetylene. They catalyze an isotopic exchange reaction between C₂H₂ and C₂D₂, significantly accelerating the rate compared to the formation of C₄H₂ []. This isotopic exchange provides evidence for the involvement of hydrogen atoms in the early stages of Acetylene decomposition.
Disclaimer and Information on In-Vitro Research Products
Please be aware that all articles and product information presented on BenchChem are intended solely for informational purposes. The products available for purchase on BenchChem are specifically designed for in-vitro studies, which are conducted outside of living organisms. In-vitro studies, derived from the Latin term "in glass," involve experiments performed in controlled laboratory settings using cells or tissues. It is important to note that these products are not categorized as medicines or drugs, and they have not received approval from the FDA for the prevention, treatment, or cure of any medical condition, ailment, or disease. We must emphasize that any form of bodily introduction of these products into humans or animals is strictly prohibited by law. It is essential to adhere to these guidelines to ensure compliance with legal and ethical standards in research and experimentation.