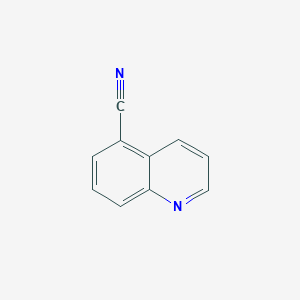
5-Cyanoquinoline
Overview
Description
5-Cyanoquinoline is a useful research compound. Its molecular formula is C10H6N2 and its molecular weight is 154.17 g/mol. The purity is usually 95%.
The exact mass of the compound this compound is unknown and the complexity rating of the compound is unknown. The United Nations designated GHS hazard class pictogram is Irritant, and the GHS signal word is WarningThe storage condition is unknown. Please store according to label instructions upon receipt of goods.
BenchChem offers high-quality this compound suitable for many research applications. Different packaging options are available to accommodate customers' requirements. Please inquire for more information about this compound including the price, delivery time, and more detailed information at info@benchchem.com.
Mechanism of Action
Target of Action
Quinoline-5-carbonitrile, also known as 5-Cyanoquinoline, is a derivative of the quinoline scaffold, which is an important construction motif for the development of new drugs . Quinoline derivatives have been reported to show significant results through different mechanisms, including the inhibition of tumor growth by cell cycle arrest, apoptosis, inhibition of angiogenesis, disruption of cell migration, and modulation of nuclear receptor responsiveness .
Mode of Action
Quinoline derivatives, in general, are known to interact with their targets, leading to various changes such as cell cycle arrest, apoptosis, inhibition of angiogenesis, disruption of cell migration, and modulation of nuclear receptor responsiveness . These interactions and resulting changes contribute to their diverse spectrum of biological activities.
Biochemical Pathways
Quinoline derivatives are known to affect various biochemical pathways, leading to a wide range of downstream effects . These effects include antifungal, anti-inflammatory, anti-diabetes, anti-Alzheimer’s disease, antioxidant, and diuretic activities .
Pharmacokinetics
Quinoline derivatives are known to have diverse pharmacokinetic properties . The structural diversity of these compounds provides high and selective activity attained through different mechanisms of action, as well as low toxicity on human cells .
Result of Action
Quinoline derivatives are known to have a wide range of biological activities, including antifungal, anti-inflammatory, anti-diabetes, anti-alzheimer’s disease, antioxidant, and diuretic activities . These activities result from the compound’s interaction with its targets and the subsequent changes in cellular processes .
Action Environment
It is known that the synthesis of quinoline derivatives can be influenced by various factors, including temperature, solvent conditions, and the presence of catalysts . These factors could potentially influence the action and stability of the compound.
Biochemical Analysis
Biochemical Properties
Quinoline-5-carbonitrile, like other quinoline derivatives, is known to interact with various enzymes, proteins, and other biomolecules. For instance, quinoline derivatives have been reported to inhibit tumor growth by cell cycle arrest, apoptosis, inhibition of angiogenesis, disruption of cell migration and modulation of nuclear receptor responsiveness
Cellular Effects
Quinoline-5-carbonitrile, similar to other quinoline derivatives, may have significant effects on various types of cells and cellular processes. Quinoline derivatives have been reported to have antiproliferative properties, potentially inhibiting tumor growth by affecting cell signaling pathways, gene expression, and cellular metabolism
Molecular Mechanism
Quinoline derivatives have been reported to exert their effects at the molecular level through various mechanisms, including binding interactions with biomolecules, enzyme inhibition or activation, and changes in gene expression
Properties
IUPAC Name |
quinoline-5-carbonitrile | |
---|---|---|
Source | PubChem | |
URL | https://pubchem.ncbi.nlm.nih.gov | |
Description | Data deposited in or computed by PubChem | |
InChI |
InChI=1S/C10H6N2/c11-7-8-3-1-5-10-9(8)4-2-6-12-10/h1-6H | |
Source | PubChem | |
URL | https://pubchem.ncbi.nlm.nih.gov | |
Description | Data deposited in or computed by PubChem | |
InChI Key |
CKKBFRWSTPFAHR-UHFFFAOYSA-N | |
Source | PubChem | |
URL | https://pubchem.ncbi.nlm.nih.gov | |
Description | Data deposited in or computed by PubChem | |
Canonical SMILES |
C1=CC(=C2C=CC=NC2=C1)C#N | |
Source | PubChem | |
URL | https://pubchem.ncbi.nlm.nih.gov | |
Description | Data deposited in or computed by PubChem | |
Molecular Formula |
C10H6N2 | |
Source | PubChem | |
URL | https://pubchem.ncbi.nlm.nih.gov | |
Description | Data deposited in or computed by PubChem | |
DSSTOX Substance ID |
DTXSID20405816 | |
Record name | 5-Cyanoquinoline | |
Source | EPA DSSTox | |
URL | https://comptox.epa.gov/dashboard/DTXSID20405816 | |
Description | DSSTox provides a high quality public chemistry resource for supporting improved predictive toxicology. | |
Molecular Weight |
154.17 g/mol | |
Source | PubChem | |
URL | https://pubchem.ncbi.nlm.nih.gov | |
Description | Data deposited in or computed by PubChem | |
CAS No. |
59551-02-9 | |
Record name | 5-Cyanoquinoline | |
Source | EPA DSSTox | |
URL | https://comptox.epa.gov/dashboard/DTXSID20405816 | |
Description | DSSTox provides a high quality public chemistry resource for supporting improved predictive toxicology. | |
Record name | quinoline-5-carbonitrile | |
Source | European Chemicals Agency (ECHA) | |
URL | https://echa.europa.eu/information-on-chemicals | |
Description | The European Chemicals Agency (ECHA) is an agency of the European Union which is the driving force among regulatory authorities in implementing the EU's groundbreaking chemicals legislation for the benefit of human health and the environment as well as for innovation and competitiveness. | |
Explanation | Use of the information, documents and data from the ECHA website is subject to the terms and conditions of this Legal Notice, and subject to other binding limitations provided for under applicable law, the information, documents and data made available on the ECHA website may be reproduced, distributed and/or used, totally or in part, for non-commercial purposes provided that ECHA is acknowledged as the source: "Source: European Chemicals Agency, http://echa.europa.eu/". Such acknowledgement must be included in each copy of the material. ECHA permits and encourages organisations and individuals to create links to the ECHA website under the following cumulative conditions: Links can only be made to webpages that provide a link to the Legal Notice page. | |
Synthesis routes and methods I
Procedure details
Synthesis routes and methods II
Procedure details
Retrosynthesis Analysis
AI-Powered Synthesis Planning: Our tool employs the Template_relevance Pistachio, Template_relevance Bkms_metabolic, Template_relevance Pistachio_ringbreaker, Template_relevance Reaxys, Template_relevance Reaxys_biocatalysis model, leveraging a vast database of chemical reactions to predict feasible synthetic routes.
One-Step Synthesis Focus: Specifically designed for one-step synthesis, it provides concise and direct routes for your target compounds, streamlining the synthesis process.
Accurate Predictions: Utilizing the extensive PISTACHIO, BKMS_METABOLIC, PISTACHIO_RINGBREAKER, REAXYS, REAXYS_BIOCATALYSIS database, our tool offers high-accuracy predictions, reflecting the latest in chemical research and data.
Strategy Settings
Precursor scoring | Relevance Heuristic |
---|---|
Min. plausibility | 0.01 |
Model | Template_relevance |
Template Set | Pistachio/Bkms_metabolic/Pistachio_ringbreaker/Reaxys/Reaxys_biocatalysis |
Top-N result to add to graph | 6 |
Feasible Synthetic Routes
Q1: What are some of the common synthetic applications of quinoline-5-carbonitrile?
A1: Quinoline-5-carbonitrile serves as a starting point for the synthesis of various heterocyclic systems with potential biological activity. For example, it is used to create:
- 1,3-Diamino-7,8,9,10-tetrahydropyrido[3,2-f]quinazolines: These compounds are inhibitors of Candida albicans dihydrofolate reductase, a potential target for antifungal agents [].
- 5-Thiocarbomoyl- and 5-(thiazol-2-yl)-2,3,4,4a,5,6-hexahydro-1H-pyrido[1,2-a]quinoline-5-carbonitriles: These compounds are synthesized through tert-amino effect cyclizations, demonstrating the versatility of quinoline-5-carbonitrile in ring-forming reactions [].
Q2: Can you elaborate on the reaction of 6-nitroquinoline with potassium cyanide and nitroalkanes, and how does quinoline-5-carbonitrile fit in?
A2: This reaction involves an intriguing aromatic nucleophilic substitution of hydrogen in the 6-nitroquinoline molecule []. Interestingly, the reaction with potassium cyanide and methyl nitroacetate leads to the formation of 6-(methoxalylamino)quinoline-5-carbonitrile, showcasing the introduction of the cyano group at the 5-position [].
Q3: Are there any structural insights available for compounds derived from quinoline-5-carbonitrile?
A3: Yes, X-ray crystallography has provided valuable information about the three-dimensional structure of derivatives like 6-(2-nitroprop-2-yl)quinoline-5-carbonitrile []. This compound, unexpectedly obtained as a minor product in the reaction of 6-nitroquinoline with 2-nitropropane and potassium cyanide, displays a cis orientation of the nitro group in the 2-nitropropyl substituent with respect to the aromatic ring []. This information provides valuable insights into the conformational preferences of these molecules.
Q4: How does the structure of quinoline-5-carbonitrile relate to its reactivity?
A4: The presence of both the electron-rich quinoline ring and the electron-withdrawing cyano group makes this compound highly versatile in chemical reactions. The quinoline nitrogen can participate in coordination chemistry, while the cyano group can be readily transformed into other functional groups, including carboxylic acids and amines.
Q5: Has quinoline-5-carbonitrile been used in the synthesis of any other noteworthy compounds?
A5: Yes, one interesting example is its incorporation into the structure of 6‐(4‐Chlorophenyl)‐9,9‐dimethyl‐3,7‐dioxo‐2,3,4,6,7,8,9,10‐octahydro‐1H-pyrimido[1,2‐a]quinoline‐5‐carbonitrile []. This complex molecule, synthesized using microwave irradiation, highlights the ability of quinoline-5-carbonitrile to participate in multi-step synthetic sequences.
Q6: Are there any known applications of quinoline-5-carbonitrile derivatives in medicinal chemistry?
A6: While specific details are limited in the provided research, the synthesis of 1,3-diamino-7,8,9,10-tetrahydropyrido[3,2-f]quinazolines as Candida albicans dihydrofolate reductase inhibitors suggests a potential role in antifungal drug development []. Further research in this area is crucial to fully understand the therapeutic potential of these compounds.
Q7: What analytical techniques are typically employed to characterize quinoline-5-carbonitrile and its derivatives?
A7: Researchers rely on various spectroscopic and analytical methods to study these compounds. NMR spectroscopy (both 1H and 13C) is crucial for structural elucidation, while techniques like mass spectrometry confirm molecular weight and provide information about fragmentation patterns [, , ]. X-ray crystallography, when applicable, offers valuable insights into three-dimensional structure [].
Disclaimer and Information on In-Vitro Research Products
Please be aware that all articles and product information presented on BenchChem are intended solely for informational purposes. The products available for purchase on BenchChem are specifically designed for in-vitro studies, which are conducted outside of living organisms. In-vitro studies, derived from the Latin term "in glass," involve experiments performed in controlled laboratory settings using cells or tissues. It is important to note that these products are not categorized as medicines or drugs, and they have not received approval from the FDA for the prevention, treatment, or cure of any medical condition, ailment, or disease. We must emphasize that any form of bodily introduction of these products into humans or animals is strictly prohibited by law. It is essential to adhere to these guidelines to ensure compliance with legal and ethical standards in research and experimentation.