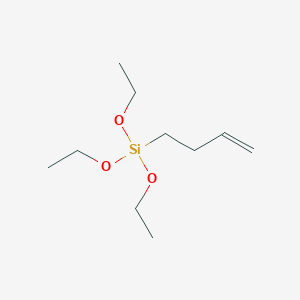
3-Butenyltriethoxysilane
Overview
Description
3-Butenyltriethoxysilane: is an organosilicon compound with the molecular formula C10H22O3Si . It is a clear, colorless liquid that is primarily used as a coupling agent and adhesion promoter in various industrial applications. The compound contains a butenyl group attached to a silicon atom, which is further bonded to three ethoxy groups. This unique structure allows it to form strong bonds with both organic and inorganic materials, making it highly valuable in the production of advanced materials and coatings.
Scientific Research Applications
3-Butenyltriethoxysilane has a wide range of applications in scientific research, including:
Chemistry: Used as a coupling agent in the synthesis of advanced materials, such as hybrid organic-inorganic polymers and nanocomposites.
Biology: Employed in the functionalization of biomolecules and surfaces for biosensor development and bioimaging.
Medicine: Utilized in drug delivery systems and the development of biocompatible coatings for medical devices.
Industry: Applied in the production of adhesives, sealants, and coatings to enhance adhesion and durability.
Mechanism of Action
Target of Action
3-Butenyltriethoxysilane is primarily used as a chemical intermediate . It is a type of organoethoxysilane , which are compounds that contain a silicon atom bonded to an ethoxy group and a hydrocarbon group. These compounds are often used in the production of silicones, a diverse family of synthetic polymers with a wide range of industrial applications.
Mode of Action
The mode of action of this compound is largely based on its chemical structure. As an organoethoxysilane, it can undergo hydrolysis to form silanols, which can then condense to form siloxane bonds . This process is fundamental to the formation of silicone polymers. The presence of the 3-butenyl group in this compound may also influence its reactivity and the properties of the resulting polymers.
Action Environment
The action of this compound can be influenced by various environmental factors. For example, the hydrolysis and condensation reactions it undergoes are dependent on conditions such as temperature and pH . Additionally, the presence of moisture can accelerate the hydrolysis reaction .
Preparation Methods
Synthetic Routes and Reaction Conditions: 3-Butenyltriethoxysilane can be synthesized through a hydrosilylation reaction, where an alkene (butene) reacts with a hydrosilane (triethoxysilane) in the presence of a platinum catalyst. The reaction typically occurs under mild conditions, with temperatures ranging from 25°C to 100°C and pressures from 1 to 5 atmospheres. The reaction can be represented as follows:
CH2=CH−CH2−CH3+H−Si(OCH2CH3)3→CH2=CH−CH2−CH2−Si(OCH2CH3)3
Industrial Production Methods: In industrial settings, this compound is produced using large-scale hydrosilylation reactors. The process involves continuous feeding of the reactants and catalyst into the reactor, where the reaction takes place under controlled conditions. The product is then purified through distillation to remove any unreacted starting materials and by-products.
Chemical Reactions Analysis
Types of Reactions: 3-Butenyltriethoxysilane undergoes various chemical reactions, including:
Hydrolysis: In the presence of water, the ethoxy groups are hydrolyzed to form silanol groups, which can further condense to form siloxane bonds.
Oxidation: The butenyl group can be oxidized to form epoxides or other oxygen-containing functional groups.
Substitution: The ethoxy groups can be substituted with other alkoxy or functional groups through nucleophilic substitution reactions.
Common Reagents and Conditions:
Hydrolysis: Water or aqueous acid/base solutions at room temperature.
Oxidation: Oxidizing agents such as hydrogen peroxide or peracids under mild conditions.
Substitution: Nucleophiles such as alcohols or amines in the presence of a catalyst.
Major Products:
Hydrolysis: Silanols and siloxanes.
Oxidation: Epoxides and hydroxylated derivatives.
Substitution: Alkoxy-substituted silanes and functionalized silanes.
Comparison with Similar Compounds
Vinyltriethoxysilane: Contains a vinyl group instead of a butenyl group, used in similar applications but with different reactivity.
Methacryloxypropyltrimethoxysilane: Contains a methacryloxy group, used in polymerization reactions and surface treatments.
Aminopropyltriethoxysilane: Contains an amino group, used for surface modification and as a coupling agent in composites.
Uniqueness: 3-Butenyltriethoxysilane is unique due to its butenyl group, which provides distinct reactivity and allows for specific functionalization that is not possible with other similar compounds. Its ability to form strong bonds with both organic and inorganic materials makes it highly versatile and valuable in various applications.
Properties
IUPAC Name |
but-3-enyl(triethoxy)silane | |
---|---|---|
Source | PubChem | |
URL | https://pubchem.ncbi.nlm.nih.gov | |
Description | Data deposited in or computed by PubChem | |
InChI |
InChI=1S/C10H22O3Si/c1-5-9-10-14(11-6-2,12-7-3)13-8-4/h5H,1,6-10H2,2-4H3 | |
Source | PubChem | |
URL | https://pubchem.ncbi.nlm.nih.gov | |
Description | Data deposited in or computed by PubChem | |
InChI Key |
LUZRKMGMNFOSFZ-UHFFFAOYSA-N | |
Source | PubChem | |
URL | https://pubchem.ncbi.nlm.nih.gov | |
Description | Data deposited in or computed by PubChem | |
Canonical SMILES |
CCO[Si](CCC=C)(OCC)OCC | |
Source | PubChem | |
URL | https://pubchem.ncbi.nlm.nih.gov | |
Description | Data deposited in or computed by PubChem | |
Molecular Formula |
C10H22O3Si | |
Source | PubChem | |
URL | https://pubchem.ncbi.nlm.nih.gov | |
Description | Data deposited in or computed by PubChem | |
DSSTOX Substance ID |
DTXSID10451746 | |
Record name | 3-BUTENYLTRIETHOXYSILANE | |
Source | EPA DSSTox | |
URL | https://comptox.epa.gov/dashboard/DTXSID10451746 | |
Description | DSSTox provides a high quality public chemistry resource for supporting improved predictive toxicology. | |
Molecular Weight |
218.36 g/mol | |
Source | PubChem | |
URL | https://pubchem.ncbi.nlm.nih.gov | |
Description | Data deposited in or computed by PubChem | |
CAS No. |
57813-67-9 | |
Record name | 3-Butenyltriethoxysilane | |
Source | CAS Common Chemistry | |
URL | https://commonchemistry.cas.org/detail?cas_rn=57813-67-9 | |
Description | CAS Common Chemistry is an open community resource for accessing chemical information. Nearly 500,000 chemical substances from CAS REGISTRY cover areas of community interest, including common and frequently regulated chemicals, and those relevant to high school and undergraduate chemistry classes. This chemical information, curated by our expert scientists, is provided in alignment with our mission as a division of the American Chemical Society. | |
Explanation | The data from CAS Common Chemistry is provided under a CC-BY-NC 4.0 license, unless otherwise stated. | |
Record name | 3-BUTENYLTRIETHOXYSILANE | |
Source | EPA DSSTox | |
URL | https://comptox.epa.gov/dashboard/DTXSID10451746 | |
Description | DSSTox provides a high quality public chemistry resource for supporting improved predictive toxicology. | |
Synthesis routes and methods
Procedure details
Retrosynthesis Analysis
AI-Powered Synthesis Planning: Our tool employs the Template_relevance Pistachio, Template_relevance Bkms_metabolic, Template_relevance Pistachio_ringbreaker, Template_relevance Reaxys, Template_relevance Reaxys_biocatalysis model, leveraging a vast database of chemical reactions to predict feasible synthetic routes.
One-Step Synthesis Focus: Specifically designed for one-step synthesis, it provides concise and direct routes for your target compounds, streamlining the synthesis process.
Accurate Predictions: Utilizing the extensive PISTACHIO, BKMS_METABOLIC, PISTACHIO_RINGBREAKER, REAXYS, REAXYS_BIOCATALYSIS database, our tool offers high-accuracy predictions, reflecting the latest in chemical research and data.
Strategy Settings
Precursor scoring | Relevance Heuristic |
---|---|
Min. plausibility | 0.01 |
Model | Template_relevance |
Template Set | Pistachio/Bkms_metabolic/Pistachio_ringbreaker/Reaxys/Reaxys_biocatalysis |
Top-N result to add to graph | 6 |
Feasible Synthetic Routes
Q1: How does the incorporation of an adamantyl group influence the properties of the coating film derived from 4-(1-Adamantyl)-3-Butenyltriethoxysilane?
A1: The study demonstrates that the presence of the bulky adamantyl group in 4-(1-Adamantyl)-3-Butenyltriethoxysilane significantly impacts the resulting coating film's properties []. The researchers observed a notable increase in film thickness compared to coatings derived from alkoxysilanes lacking this group. This suggests that the adamantyl group influences the packing and crosslinking density during film formation. Additionally, the adamantyl group contributes to enhanced hardness, indicating improved mechanical properties of the coating. While the adamantyl group doesn't dramatically alter the water repellent nature compared to a methyl group, it still yields a coating with notable hydrophobicity.
Disclaimer and Information on In-Vitro Research Products
Please be aware that all articles and product information presented on BenchChem are intended solely for informational purposes. The products available for purchase on BenchChem are specifically designed for in-vitro studies, which are conducted outside of living organisms. In-vitro studies, derived from the Latin term "in glass," involve experiments performed in controlled laboratory settings using cells or tissues. It is important to note that these products are not categorized as medicines or drugs, and they have not received approval from the FDA for the prevention, treatment, or cure of any medical condition, ailment, or disease. We must emphasize that any form of bodily introduction of these products into humans or animals is strictly prohibited by law. It is essential to adhere to these guidelines to ensure compliance with legal and ethical standards in research and experimentation.