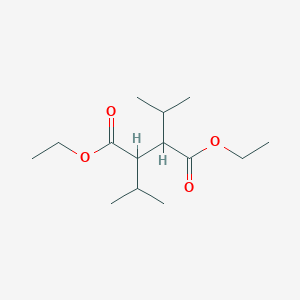
Diethyl 2,3-diisopropylsuccinate
Overview
Description
Diethyl 2,3-diisopropylsuccinate is an organic compound primarily used as an intermediate in the production of fine chemicals and spices. It is also utilized in the synthesis of various perfumes, flavors, and food additives . This compound is known for its role as an internal donor in the production of polypropylene, a widely used plastic.
Mechanism of Action
- Its role is to enhance stereospecificity and regulate the polymerization process during polypropylene synthesis .
- This interaction affects the polymerization of propylene, leading to the formation of polypropylene with a broad molecular-mass distribution .
- Through this pathway, it contributes to the broad molecular-mass distribution of the resulting polymer .
- Its ADME (absorption, distribution, metabolism, and excretion) properties impact its bioavailability and subsequent use in polypropylene production .
Target of Action
Mode of Action
Biochemical Pathways
Pharmacokinetics
Result of Action
Action Environment
Biochemical Analysis
Biochemical Properties
Diethyl 2,3-diisopropylsuccinate plays a significant role in biochemical reactions, particularly in the synthesis of stereoregulating components for titanium–magnesium catalysts. These catalysts are crucial for the polymerization of propylene to produce polypropylene with high isotacticity. The compound interacts with titanium and magnesium ions, forming complexes that regulate the stereochemistry of the polymerization process. This interaction ensures the production of polypropylene with a high degree of stereoregularity and broad molecular-mass distribution .
Cellular Effects
The effects of this compound on various types of cells and cellular processes are not extensively documented. Its role in the synthesis of stereoregulating components suggests that it may influence cell function indirectly through its impact on polymerization processes. The compound’s interaction with titanium and magnesium ions could potentially affect cell signaling pathways, gene expression, and cellular metabolism, although specific studies on these effects are limited .
Molecular Mechanism
This compound exerts its effects at the molecular level by forming complexes with titanium and magnesium ions. These complexes act as stereoregulating components in titanium–magnesium catalysts, which are used in the polymerization of propylene. The binding interactions between this compound and the metal ions ensure the production of polypropylene with high isotacticity. This process involves the coordination of the compound with the metal ions, which regulates the stereochemistry of the polymerization reaction .
Temporal Effects in Laboratory Settings
In laboratory settings, the stability and degradation of this compound are crucial factors that influence its effectiveness as a stereoregulating component. The compound is generally stable under standard conditions, but its long-term effects on cellular function have not been extensively studied. In in vitro and in vivo studies, the compound’s stability and degradation over time can impact its ability to form effective complexes with titanium and magnesium ions, thereby affecting the polymerization process .
Metabolic Pathways
This compound is involved in metabolic pathways related to the synthesis of stereoregulating components for titanium–magnesium catalysts. The compound interacts with enzymes and cofactors that facilitate its conversion into active complexes with titanium and magnesium ions. These interactions are crucial for regulating the stereochemistry of the polymerization process and ensuring the production of high-quality polypropylene .
Transport and Distribution
The transport and distribution of this compound within cells and tissues are influenced by its interaction with transporters and binding proteins. The compound’s ability to form complexes with titanium and magnesium ions suggests that it may be transported and distributed in a manner that facilitates its role as a stereoregulating component. This distribution is essential for ensuring the compound’s effectiveness in the polymerization process .
Subcellular Localization
The subcellular localization of this compound is likely influenced by its interaction with targeting signals and post-translational modifications. These interactions direct the compound to specific compartments or organelles where it can form effective complexes with titanium and magnesium ions. This localization is crucial for regulating the stereochemistry of the polymerization process and ensuring the production of high-quality polypropylene .
Preparation Methods
Synthetic Routes and Reaction Conditions
Diethyl 2,3-diisopropylsuccinate can be synthesized through a multi-step reaction process. The synthesis involves the condensation of a succinic acid diester with two isobutyraldehyde molecules, followed by esterification and hydrogenation of the resulting dienes . Another method involves the reaction of diethyl succinate with 2,3-diisopropyl alcohol under acid catalysis . The reaction conditions typically include the use of ethanol and potassium under an inert atmosphere, followed by reflux and treatment with sulfuric acid and water .
Industrial Production Methods
In industrial settings, the production of this compound involves similar synthetic routes but on a larger scale. The process is optimized to achieve high yields and purity, often exceeding 95% . The compound is produced in facilities equipped with the necessary reactors and purification systems to ensure consistent quality.
Chemical Reactions Analysis
Types of Reactions
Diethyl 2,3-diisopropylsuccinate undergoes various chemical reactions, including:
Oxidation: The compound can be oxidized to form corresponding carboxylic acids.
Reduction: Reduction reactions can convert it into alcohols or other reduced forms.
Substitution: It can participate in substitution reactions where functional groups are replaced by other groups.
Common Reagents and Conditions
Common reagents used in these reactions include oxidizing agents like potassium permanganate for oxidation, reducing agents like lithium aluminum hydride for reduction, and various nucleophiles for substitution reactions. The conditions vary depending on the desired reaction, but they often involve controlled temperatures and inert atmospheres to prevent unwanted side reactions.
Major Products Formed
The major products formed from these reactions depend on the specific reaction type. For example, oxidation typically yields carboxylic acids, while reduction can produce alcohols. Substitution reactions result in the formation of new compounds with different functional groups.
Scientific Research Applications
Diethyl 2,3-diisopropylsuccinate has several scientific research applications:
Biology: The compound is studied for its potential biological activities and interactions with biological molecules.
Medicine: Research is ongoing to explore its potential therapeutic applications and its role in drug synthesis.
Comparison with Similar Compounds
Diethyl 2,3-diisopropylsuccinate is compared with other similar compounds, such as diethyl 2,3-diisobutylsuccinate. Both compounds are used as internal donors in polypropylene production, but this compound is more widely used due to its superior performance and higher purity . Other similar compounds include various 2,3-dialkyl-substituted succinates, which also serve as electron-donor compounds in catalyst systems .
Conclusion
This compound is a versatile compound with significant applications in chemistry, biology, medicine, and industry. Its unique properties and effectiveness as an internal donor in polypropylene production make it a valuable compound for scientific research and industrial applications.
Properties
IUPAC Name |
diethyl 2,3-di(propan-2-yl)butanedioate | |
---|---|---|
Source | PubChem | |
URL | https://pubchem.ncbi.nlm.nih.gov | |
Description | Data deposited in or computed by PubChem | |
InChI |
InChI=1S/C14H26O4/c1-7-17-13(15)11(9(3)4)12(10(5)6)14(16)18-8-2/h9-12H,7-8H2,1-6H3 | |
Source | PubChem | |
URL | https://pubchem.ncbi.nlm.nih.gov | |
Description | Data deposited in or computed by PubChem | |
InChI Key |
WGFNXLQURMLAGC-UHFFFAOYSA-N | |
Source | PubChem | |
URL | https://pubchem.ncbi.nlm.nih.gov | |
Description | Data deposited in or computed by PubChem | |
Canonical SMILES |
CCOC(=O)C(C(C)C)C(C(C)C)C(=O)OCC | |
Source | PubChem | |
URL | https://pubchem.ncbi.nlm.nih.gov | |
Description | Data deposited in or computed by PubChem | |
Molecular Formula |
C14H26O4 | |
Source | PubChem | |
URL | https://pubchem.ncbi.nlm.nih.gov | |
Description | Data deposited in or computed by PubChem | |
DSSTOX Substance ID |
DTXSID10431240 | |
Record name | diethyl 2,3-diisopropylsuccinate | |
Source | EPA DSSTox | |
URL | https://comptox.epa.gov/dashboard/DTXSID10431240 | |
Description | DSSTox provides a high quality public chemistry resource for supporting improved predictive toxicology. | |
Molecular Weight |
258.35 g/mol | |
Source | PubChem | |
URL | https://pubchem.ncbi.nlm.nih.gov | |
Description | Data deposited in or computed by PubChem | |
CAS No. |
33367-55-4 | |
Record name | diethyl 2,3-diisopropylsuccinate | |
Source | EPA DSSTox | |
URL | https://comptox.epa.gov/dashboard/DTXSID10431240 | |
Description | DSSTox provides a high quality public chemistry resource for supporting improved predictive toxicology. | |
Record name | diethyl 2,3-di(propan-2-yl)butanedioate | |
Source | European Chemicals Agency (ECHA) | |
URL | https://echa.europa.eu/information-on-chemicals | |
Description | The European Chemicals Agency (ECHA) is an agency of the European Union which is the driving force among regulatory authorities in implementing the EU's groundbreaking chemicals legislation for the benefit of human health and the environment as well as for innovation and competitiveness. | |
Explanation | Use of the information, documents and data from the ECHA website is subject to the terms and conditions of this Legal Notice, and subject to other binding limitations provided for under applicable law, the information, documents and data made available on the ECHA website may be reproduced, distributed and/or used, totally or in part, for non-commercial purposes provided that ECHA is acknowledged as the source: "Source: European Chemicals Agency, http://echa.europa.eu/". Such acknowledgement must be included in each copy of the material. ECHA permits and encourages organisations and individuals to create links to the ECHA website under the following cumulative conditions: Links can only be made to webpages that provide a link to the Legal Notice page. | |
Retrosynthesis Analysis
AI-Powered Synthesis Planning: Our tool employs the Template_relevance Pistachio, Template_relevance Bkms_metabolic, Template_relevance Pistachio_ringbreaker, Template_relevance Reaxys, Template_relevance Reaxys_biocatalysis model, leveraging a vast database of chemical reactions to predict feasible synthetic routes.
One-Step Synthesis Focus: Specifically designed for one-step synthesis, it provides concise and direct routes for your target compounds, streamlining the synthesis process.
Accurate Predictions: Utilizing the extensive PISTACHIO, BKMS_METABOLIC, PISTACHIO_RINGBREAKER, REAXYS, REAXYS_BIOCATALYSIS database, our tool offers high-accuracy predictions, reflecting the latest in chemical research and data.
Strategy Settings
Precursor scoring | Relevance Heuristic |
---|---|
Min. plausibility | 0.01 |
Model | Template_relevance |
Template Set | Pistachio/Bkms_metabolic/Pistachio_ringbreaker/Reaxys/Reaxys_biocatalysis |
Top-N result to add to graph | 6 |
Feasible Synthetic Routes
Q1: What is the role of Diethyl 2,3-diisopropylsuccinate (DISE) in polypropylene catalysts?
A1: DISE acts as an internal electron donor in Ziegler-Natta catalysts used for polypropylene production [, , ]. These catalysts typically consist of a titanium compound supported on magnesium chloride. Internal donors like DISE influence the catalyst's activity, stereoselectivity (ability to control the arrangement of monomer units in the polymer chain), and molecular weight distribution of the produced polypropylene [, ].
Q2: How does the stereochemistry of DISE impact its performance as an internal donor?
A2: Research has shown that both enantiomers of DISE, specifically diethyl (+)-2,3-diisopropylsuccinate and its corresponding (-) enantiomer, can be effective internal donors in polypropylene catalysts []. Interestingly, despite their different molecular conformations, both enantiomers seem to confer similar performance characteristics to the catalyst in terms of activity and stereoselectivity []. This suggests that the specific spatial arrangement of the enantiomers may not be critical for their interaction with the catalyst's active sites [].
Q3: How does DISE interact with the titanium component of the Ziegler-Natta catalyst?
A3: While specific details require further investigation, calorimetric studies have provided insights into the interaction between titanium tetrachloride (TiCl4), a common precursor to the active titanium species in Ziegler-Natta catalysts, and various Lewis bases, including DISE []. These studies suggest that DISE coordinates to the TiCl4 through its carbonyl groups, forming a complex []. The strength of this interaction is influenced by both electronic and steric factors associated with the Lewis base []. This complexation likely plays a role in the formation and stabilization of the active catalytic species, ultimately influencing the polymerization process.
Q4: How does the use of DISE as an internal donor compare to other electron donors in Ziegler-Natta catalysts?
A4: Research indicates that incorporating cycloalkoxy silane compounds as external electron donors (EEDs) alongside DISE as an internal donor in Ziegler-Natta catalysts can lead to enhanced properties in the produced polypropylene []. Specifically, this combination can result in higher melt flow rates (MFR), indicating improved processability of the polymer, and lower average molecular weights compared to using a commercial EED like cyclohexyl methyl dimethoxysilane []. This highlights the importance of carefully selecting and combining both internal and external donors to fine-tune the catalyst's performance and achieve desired polymer characteristics.
Q5: Are there any analytical techniques used to study the interaction of DISE with Ziegler-Natta catalysts?
A5: Yes, diffuse reflectance infrared Fourier transform spectroscopy (DRIFTS) has been employed to study the state of various electron-donating compounds, including internal donors like DISE, within titanium-magnesium catalysts used for propylene polymerization []. This technique allows researchers to gain insights into the coordination and interaction of these compounds with the catalyst's components, providing valuable information about the catalyst's structure and behavior.
Disclaimer and Information on In-Vitro Research Products
Please be aware that all articles and product information presented on BenchChem are intended solely for informational purposes. The products available for purchase on BenchChem are specifically designed for in-vitro studies, which are conducted outside of living organisms. In-vitro studies, derived from the Latin term "in glass," involve experiments performed in controlled laboratory settings using cells or tissues. It is important to note that these products are not categorized as medicines or drugs, and they have not received approval from the FDA for the prevention, treatment, or cure of any medical condition, ailment, or disease. We must emphasize that any form of bodily introduction of these products into humans or animals is strictly prohibited by law. It is essential to adhere to these guidelines to ensure compliance with legal and ethical standards in research and experimentation.