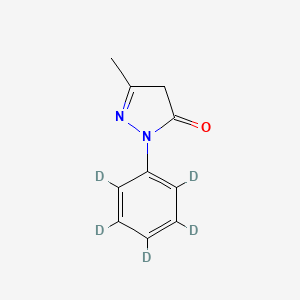
Edaravone D5
Overview
Description
Edaravone D5 is a useful research compound. Its molecular formula is C10H10N2O and its molecular weight is 179.23 g/mol. The purity is usually 95%.
BenchChem offers high-quality this compound suitable for many research applications. Different packaging options are available to accommodate customers' requirements. Please inquire for more information about this compound including the price, delivery time, and more detailed information at info@benchchem.com.
Mechanism of Action
- Edaravone scavenges and suppresses the generation of hydroxyl radicals and peroxynitrite radicals .
- By preventing oxidative damage to cell membranes, it may contribute to inhibiting the progression of ALS .
- The exact mechanism of action in ALS is not fully elucidated, but its antioxidant properties likely mediate therapeutic effects .
- Edaravone-activated neurotrophic factor signaling pathways, especially GDNF/RET signaling, have been discovered .
Target of Action
Mode of Action
Biochemical Pathways
Pharmacokinetics
Result of Action
Biochemical Analysis
Biochemical Properties
Edaravone D5 plays a crucial role in biochemical reactions by scavenging reactive oxygen species (ROS) and reducing oxidative stress. It interacts with various enzymes, proteins, and biomolecules involved in oxidative stress pathways. For instance, this compound interacts with superoxide dismutase (SOD) and catalase, enhancing their antioxidant activities. Additionally, this compound binds to peroxynitrite, a reactive nitrogen species, and neutralizes its harmful effects .
Cellular Effects
This compound exerts significant effects on various cell types and cellular processes. It has been shown to protect neurons from oxidative damage by reducing ROS levels and enhancing mitochondrial function. This compound influences cell signaling pathways, such as the nuclear factor erythroid 2-related factor 2 (Nrf2) pathway, which regulates the expression of antioxidant genes. Furthermore, this compound modulates gene expression related to oxidative stress and inflammation, thereby improving cellular metabolism and function .
Molecular Mechanism
The molecular mechanism of this compound involves its ability to scavenge free radicals and inhibit oxidative stress. This compound binds to ROS and reactive nitrogen species, neutralizing their harmful effects. It also activates the Nrf2 pathway, leading to the upregulation of antioxidant genes such as heme oxygenase-1 (HO-1) and glutathione peroxidase (GPx). Additionally, this compound inhibits the activation of pro-inflammatory transcription factors, such as nuclear factor-kappa B (NF-κB), reducing inflammation and oxidative damage .
Temporal Effects in Laboratory Settings
In laboratory settings, the effects of this compound have been observed to change over time. This compound demonstrates good stability and minimal degradation under standard laboratory conditions. Long-term studies have shown that this compound maintains its neuroprotective effects over extended periods, with consistent reduction in oxidative stress and improvement in cellular function. In both in vitro and in vivo studies, this compound has shown sustained efficacy in protecting neurons and other cell types from oxidative damage .
Dosage Effects in Animal Models
The effects of this compound vary with different dosages in animal models. At lower doses, this compound effectively reduces oxidative stress and improves motor function in animal models of neurodegenerative diseases. At higher doses, this compound may exhibit toxic effects, such as hepatotoxicity and nephrotoxicity. It is crucial to determine the optimal dosage to maximize the therapeutic benefits while minimizing adverse effects .
Metabolic Pathways
This compound is involved in several metabolic pathways, including the Sirt1/Nrf2/HO-1/GPx4 pathway. This pathway plays a critical role in regulating oxidative stress and inflammation. This compound enhances the activity of Sirt1, a deacetylase enzyme, which subsequently activates Nrf2. Activated Nrf2 translocates to the nucleus and induces the expression of antioxidant genes, such as HO-1 and GPx4. These genes help in reducing oxidative damage and improving cellular function .
Transport and Distribution
This compound is transported and distributed within cells and tissues through various mechanisms. It readily crosses the blood-brain barrier, allowing it to exert its neuroprotective effects in the central nervous system. This compound is distributed to various organs, including the heart, lung, and kidney. It is excreted equally via urine and feces. The pharmacokinetics and tissue distribution of this compound have been well-characterized in pre-clinical studies .
Subcellular Localization
This compound exhibits specific subcellular localization, which is crucial for its activity and function. It is primarily localized in the mitochondria, where it exerts its antioxidant effects by reducing mitochondrial ROS levels. Additionally, this compound can be found in the cytoplasm and nucleus, where it modulates gene expression and cell signaling pathways. The subcellular localization of this compound is influenced by targeting signals and post-translational modifications .
Properties
IUPAC Name |
5-methyl-2-(2,3,4,5,6-pentadeuteriophenyl)-4H-pyrazol-3-one | |
---|---|---|
Source | PubChem | |
URL | https://pubchem.ncbi.nlm.nih.gov | |
Description | Data deposited in or computed by PubChem | |
InChI |
InChI=1S/C10H10N2O/c1-8-7-10(13)12(11-8)9-5-3-2-4-6-9/h2-6H,7H2,1H3/i2D,3D,4D,5D,6D | |
Source | PubChem | |
URL | https://pubchem.ncbi.nlm.nih.gov | |
Description | Data deposited in or computed by PubChem | |
InChI Key |
QELUYTUMUWHWMC-VIQYUKPQSA-N | |
Source | PubChem | |
URL | https://pubchem.ncbi.nlm.nih.gov | |
Description | Data deposited in or computed by PubChem | |
Canonical SMILES |
CC1=NN(C(=O)C1)C2=CC=CC=C2 | |
Source | PubChem | |
URL | https://pubchem.ncbi.nlm.nih.gov | |
Description | Data deposited in or computed by PubChem | |
Isomeric SMILES |
[2H]C1=C(C(=C(C(=C1[2H])[2H])N2C(=O)CC(=N2)C)[2H])[2H] | |
Source | PubChem | |
URL | https://pubchem.ncbi.nlm.nih.gov | |
Description | Data deposited in or computed by PubChem | |
Molecular Formula |
C10H10N2O | |
Source | PubChem | |
URL | https://pubchem.ncbi.nlm.nih.gov | |
Description | Data deposited in or computed by PubChem | |
Molecular Weight |
179.23 g/mol | |
Source | PubChem | |
URL | https://pubchem.ncbi.nlm.nih.gov | |
Description | Data deposited in or computed by PubChem | |
Synthesis routes and methods
Procedure details
Retrosynthesis Analysis
AI-Powered Synthesis Planning: Our tool employs the Template_relevance Pistachio, Template_relevance Bkms_metabolic, Template_relevance Pistachio_ringbreaker, Template_relevance Reaxys, Template_relevance Reaxys_biocatalysis model, leveraging a vast database of chemical reactions to predict feasible synthetic routes.
One-Step Synthesis Focus: Specifically designed for one-step synthesis, it provides concise and direct routes for your target compounds, streamlining the synthesis process.
Accurate Predictions: Utilizing the extensive PISTACHIO, BKMS_METABOLIC, PISTACHIO_RINGBREAKER, REAXYS, REAXYS_BIOCATALYSIS database, our tool offers high-accuracy predictions, reflecting the latest in chemical research and data.
Strategy Settings
Precursor scoring | Relevance Heuristic |
---|---|
Min. plausibility | 0.01 |
Model | Template_relevance |
Template Set | Pistachio/Bkms_metabolic/Pistachio_ringbreaker/Reaxys/Reaxys_biocatalysis |
Top-N result to add to graph | 6 |
Feasible Synthetic Routes
Disclaimer and Information on In-Vitro Research Products
Please be aware that all articles and product information presented on BenchChem are intended solely for informational purposes. The products available for purchase on BenchChem are specifically designed for in-vitro studies, which are conducted outside of living organisms. In-vitro studies, derived from the Latin term "in glass," involve experiments performed in controlled laboratory settings using cells or tissues. It is important to note that these products are not categorized as medicines or drugs, and they have not received approval from the FDA for the prevention, treatment, or cure of any medical condition, ailment, or disease. We must emphasize that any form of bodily introduction of these products into humans or animals is strictly prohibited by law. It is essential to adhere to these guidelines to ensure compliance with legal and ethical standards in research and experimentation.