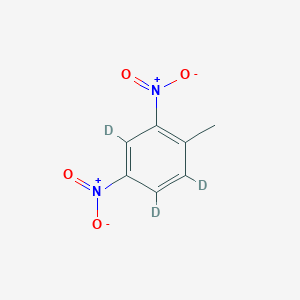
2,4-Dinitrotoluene (ring-D3)
Overview
Description
2,4-Dinitrotoluene (ring-D3) is an organic compound with the chemical formula C7H6N2O4. It is a pale yellow crystalline solid known for its use as a precursor to trinitrotoluene (TNT) and toluene diisocyanate. This compound is primarily produced through the nitration of toluene and is one of the six possible isomers of dinitrotoluene .
Mechanism of Action
Target of Action
The primary target of 2,4-Dinitrotoluene (ring-D3) is hemoglobin, a protein in red blood cells that carries oxygen . The compound interacts with hemoglobin, leading to its conversion into methemoglobin .
Mode of Action
2,4-Dinitrotoluene (ring-D3) interacts with its target, hemoglobin, through a process known as oxidative denitration . This interaction results in the conversion of hemoglobin into methemoglobin , which is unable to carry oxygen effectively, leading to a condition known as methemoglobinemia .
Biochemical Pathways
The biochemical pathway affected by 2,4-Dinitrotoluene (ring-D3) involves the conversion of hemoglobin into methemoglobin . This process disrupts the normal oxygen-carrying function of red blood cells, leading to a decrease in the amount of oxygen that reaches body tissues . Additionally, 2,4-Dinitrotoluene (ring-D3) is involved in the degradation pathways in bacteria, where it uses novel oxygenases for oxidative denitration and subsequent ring-fission .
Pharmacokinetics
The pharmacokinetics of 2,4-Dinitrotoluene (ring-D3) involve its absorption, distribution, metabolism, and excretion (ADME). The compound has a vapor pressure of 1.47X10-4 mm Hg at 22°C , indicating its potential for volatilization.
Result of Action
The primary molecular effect of 2,4-Dinitrotoluene (ring-D3) is the conversion of hemoglobin into methemoglobin . This conversion disrupts the oxygen-carrying capacity of red blood cells, leading to a condition known as methemoglobinemia . On a cellular level, this can result in a lack of oxygen in body tissues, potentially leading to cyanosis and other health effects .
Action Environment
The action of 2,4-Dinitrotoluene (ring-D3) can be influenced by various environmental factors. For instance, the compound can degrade by several mechanisms in the environment, including oxidation, photolysis, and biodegradation in water and soil . Furthermore, the compound’s action can be affected by its presence in different environmental media, such as air, food, water, and soil .
Biochemical Analysis
Biochemical Properties
2,4-Dinitrotoluene (ring-D3) plays a significant role in various biochemical reactions. It interacts with several enzymes, proteins, and other biomolecules. One of the key interactions is with oxygenases, which facilitate the oxidative denitration and subsequent ring-fission of the compound . These interactions are essential for the degradation of 2,4-Dinitrotoluene (ring-D3) in bacterial systems, highlighting its role in microbial catabolic pathways .
Cellular Effects
The effects of 2,4-Dinitrotoluene (ring-D3) on cellular processes are profound. In zebrafish, exposure to this compound has been shown to induce liver developmental damage and perturb lipid metabolism and oxygen transport gene expression . The compound influences cell function by disturbing lipid transport and metabolism, leading to severe liver damage and hypoxia in exposed organisms . Additionally, it affects cell signaling pathways and gene expression related to respiration and lipid metabolism .
Molecular Mechanism
At the molecular level, 2,4-Dinitrotoluene (ring-D3) exerts its effects through various mechanisms. It undergoes oxidative denitration facilitated by novel oxygenases, leading to the formation of intermediates that undergo further degradation . The compound’s interaction with these enzymes results in the cleavage of the aromatic ring, which is a critical step in its catabolic pathway . This process highlights the compound’s ability to influence enzyme activity and gene expression related to its degradation.
Temporal Effects in Laboratory Settings
In laboratory settings, the effects of 2,4-Dinitrotoluene (ring-D3) change over time. The compound’s stability and degradation are influenced by various factors, including environmental conditions and the presence of specific enzymes . Studies have shown that prolonged exposure to 2,4-Dinitrotoluene (ring-D3) can lead to significant changes in cellular function, including alterations in lipid metabolism and oxygen transport . These long-term effects are crucial for understanding the compound’s impact on biological systems.
Dosage Effects in Animal Models
The effects of 2,4-Dinitrotoluene (ring-D3) vary with different dosages in animal models. In bullfrogs, the compound has been shown to cause acute toxicity at high doses, with symptoms including changes in skin color, body weight, seizures, and organ necrosis . The median lethal dose (LD50) for 2,4-Dinitrotoluene (ring-D3) in bullfrogs is approximately 1,098 mg/kg body weight . These findings highlight the importance of dosage in determining the compound’s toxic and adverse effects.
Metabolic Pathways
2,4-Dinitrotoluene (ring-D3) is involved in several metabolic pathways, particularly in bacterial systems. The compound undergoes oxidative denitration and subsequent ring-fission, facilitated by specific oxygenases . These metabolic pathways are crucial for the degradation of 2,4-Dinitrotoluene (ring-D3) and its conversion into less harmful intermediates . The involvement of enzymes such as oxygenases and dehydrogenases underscores the compound’s role in microbial catabolism.
Transport and Distribution
The transport and distribution of 2,4-Dinitrotoluene (ring-D3) within cells and tissues are influenced by its interactions with transporters and binding proteins. In zebrafish, the compound has been shown to disturb lipid transport and metabolism, leading to its accumulation in liver tissues . This accumulation can result in severe liver damage and hypoxia, highlighting the importance of understanding the compound’s distribution within biological systems .
Subcellular Localization
The subcellular localization of 2,4-Dinitrotoluene (ring-D3) plays a crucial role in its activity and function. The compound’s localization within specific cellular compartments, such as the cytosol and mitochondria, can influence its interactions with enzymes and other biomolecules . Understanding the targeting signals and post-translational modifications that direct 2,4-Dinitrotoluene (ring-D3) to specific compartments is essential for elucidating its biochemical effects.
Preparation Methods
Synthetic Routes and Reaction Conditions
2,4-Dinitrotoluene is synthesized through the nitration of toluene using a mixture of concentrated nitric acid and sulfuric acid. The nitration process involves the sequential addition of nitro groups to the toluene molecule. The reaction conditions typically include maintaining a temperature range of 30-60°C to control the rate of nitration and prevent the formation of unwanted by-products .
Industrial Production Methods
In industrial settings, the production of 2,4-Dinitrotoluene involves a continuous nitration process where toluene is reacted with mixed acid (a combination of nitric acid and sulfuric acid) in large reactors. The reaction mixture is then separated, and the 2,4-Dinitrotoluene is purified through crystallization and distillation .
Chemical Reactions Analysis
Types of Reactions
2,4-Dinitrotoluene undergoes various chemical reactions, including:
Substitution: Nucleophilic substitution reactions can occur, where the nitro groups are replaced by other functional groups.
Common Reagents and Conditions
Oxidation: Fenton’s reagent (hydrogen peroxide and ferrous iron) is commonly used under acidic conditions.
Reduction: Hydrogen gas in the presence of a metal catalyst such as palladium or platinum is used for hydrogenation.
Substitution: Nucleophiles like hydroxide ions or amines can be used under basic conditions.
Major Products Formed
Oxidation: Carboxylic acids and other oxidized derivatives.
Reduction: 2,4-Diaminotoluene.
Substitution: Various substituted aromatic compounds depending on the nucleophile used.
Scientific Research Applications
2,4-Dinitrotoluene has several scientific research applications:
Comparison with Similar Compounds
Similar Compounds
2,6-Dinitrotoluene: Another isomer of dinitrotoluene with similar chemical properties but different reactivity and applications.
2,4,6-Trinitrotoluene (TNT): A highly explosive compound derived from 2,4-Dinitrotoluene through further nitration.
Nitrobenzene: A simpler nitroaromatic compound used in the synthesis of aniline and other chemicals.
Uniqueness
2,4-Dinitrotoluene is unique due to its specific position of nitro groups on the aromatic ring, which influences its reactivity and applications. It serves as a crucial intermediate in the production of both explosives and polyurethane precursors, making it a versatile compound in various industrial processes .
Properties
IUPAC Name |
1,2,5-trideuterio-3-methyl-4,6-dinitrobenzene | |
---|---|---|
Source | PubChem | |
URL | https://pubchem.ncbi.nlm.nih.gov | |
Description | Data deposited in or computed by PubChem | |
InChI |
InChI=1S/C7H6N2O4/c1-5-2-3-6(8(10)11)4-7(5)9(12)13/h2-4H,1H3/i2D,3D,4D | |
Source | PubChem | |
URL | https://pubchem.ncbi.nlm.nih.gov | |
Description | Data deposited in or computed by PubChem | |
InChI Key |
RMBFBMJGBANMMK-NRUYWUNFSA-N | |
Source | PubChem | |
URL | https://pubchem.ncbi.nlm.nih.gov | |
Description | Data deposited in or computed by PubChem | |
Canonical SMILES |
CC1=C(C=C(C=C1)[N+](=O)[O-])[N+](=O)[O-] | |
Source | PubChem | |
URL | https://pubchem.ncbi.nlm.nih.gov | |
Description | Data deposited in or computed by PubChem | |
Isomeric SMILES |
[2H]C1=C(C(=C(C(=C1C)[N+](=O)[O-])[2H])[N+](=O)[O-])[2H] | |
Source | PubChem | |
URL | https://pubchem.ncbi.nlm.nih.gov | |
Description | Data deposited in or computed by PubChem | |
Molecular Formula |
C7H6N2O4 | |
Source | PubChem | |
URL | https://pubchem.ncbi.nlm.nih.gov | |
Description | Data deposited in or computed by PubChem | |
DSSTOX Substance ID |
DTXSID501279885 | |
Record name | Benzene-1,2,4-d3, 6-methyl-3,5-dinitro- | |
Source | EPA DSSTox | |
URL | https://comptox.epa.gov/dashboard/DTXSID501279885 | |
Description | DSSTox provides a high quality public chemistry resource for supporting improved predictive toxicology. | |
Molecular Weight |
185.15 g/mol | |
Source | PubChem | |
URL | https://pubchem.ncbi.nlm.nih.gov | |
Description | Data deposited in or computed by PubChem | |
CAS No. |
93951-68-9 | |
Record name | Benzene-1,2,4-d3, 6-methyl-3,5-dinitro- | |
Source | CAS Common Chemistry | |
URL | https://commonchemistry.cas.org/detail?cas_rn=93951-68-9 | |
Description | CAS Common Chemistry is an open community resource for accessing chemical information. Nearly 500,000 chemical substances from CAS REGISTRY cover areas of community interest, including common and frequently regulated chemicals, and those relevant to high school and undergraduate chemistry classes. This chemical information, curated by our expert scientists, is provided in alignment with our mission as a division of the American Chemical Society. | |
Explanation | The data from CAS Common Chemistry is provided under a CC-BY-NC 4.0 license, unless otherwise stated. | |
Record name | Benzene-1,2,4-d3, 6-methyl-3,5-dinitro- | |
Source | EPA DSSTox | |
URL | https://comptox.epa.gov/dashboard/DTXSID501279885 | |
Description | DSSTox provides a high quality public chemistry resource for supporting improved predictive toxicology. | |
Synthesis routes and methods I
Procedure details
Synthesis routes and methods II
Procedure details
Retrosynthesis Analysis
AI-Powered Synthesis Planning: Our tool employs the Template_relevance Pistachio, Template_relevance Bkms_metabolic, Template_relevance Pistachio_ringbreaker, Template_relevance Reaxys, Template_relevance Reaxys_biocatalysis model, leveraging a vast database of chemical reactions to predict feasible synthetic routes.
One-Step Synthesis Focus: Specifically designed for one-step synthesis, it provides concise and direct routes for your target compounds, streamlining the synthesis process.
Accurate Predictions: Utilizing the extensive PISTACHIO, BKMS_METABOLIC, PISTACHIO_RINGBREAKER, REAXYS, REAXYS_BIOCATALYSIS database, our tool offers high-accuracy predictions, reflecting the latest in chemical research and data.
Strategy Settings
Precursor scoring | Relevance Heuristic |
---|---|
Min. plausibility | 0.01 |
Model | Template_relevance |
Template Set | Pistachio/Bkms_metabolic/Pistachio_ringbreaker/Reaxys/Reaxys_biocatalysis |
Top-N result to add to graph | 6 |
Feasible Synthetic Routes
Disclaimer and Information on In-Vitro Research Products
Please be aware that all articles and product information presented on BenchChem are intended solely for informational purposes. The products available for purchase on BenchChem are specifically designed for in-vitro studies, which are conducted outside of living organisms. In-vitro studies, derived from the Latin term "in glass," involve experiments performed in controlled laboratory settings using cells or tissues. It is important to note that these products are not categorized as medicines or drugs, and they have not received approval from the FDA for the prevention, treatment, or cure of any medical condition, ailment, or disease. We must emphasize that any form of bodily introduction of these products into humans or animals is strictly prohibited by law. It is essential to adhere to these guidelines to ensure compliance with legal and ethical standards in research and experimentation.