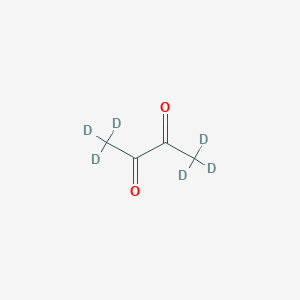
2,3-Butanedione-D6
Overview
Description
It is a stable isotope-labeled compound with the molecular formula C4D6O2 and a molecular weight of 92.13 g/mol . This compound is commonly used in scientific research due to its unique properties, which include the presence of deuterium atoms that replace hydrogen atoms in the molecule.
Scientific Research Applications
2,3-Butanedione-D6 has a wide range of applications in scientific research:
Safety and Hazards
Future Directions
Mechanism of Action
Target of Action
The primary target of 2,3-Butanedione-D6 is the enzyme myosin ATPase . Myosin ATPase is a crucial enzyme involved in muscle contraction and other cellular processes that require movement. It catalyzes the hydrolysis of ATP to generate energy for these processes .
Mode of Action
This compound functions as an inhibitor of enzymes participating in metabolic pathways by binding to the enzyme’s active site, thereby stopping the enzyme from catalyzing the reaction . Specifically, it acts as a noncompetitive inhibitor of myosin ATPase . This inhibition allows for the study of the enzyme’s kinetics and the metabolic pathways and intermediates implicated in the reaction .
Biochemical Pathways
The inhibition of myosin ATPase by this compound affects the actin-myosin interaction, a fundamental process in muscle contraction . This disruption can lead to changes in cell shape and polarity, as well as modulate the paracellular pathway in epithelial cells . Furthermore, it can influence the regulation of ion and water channel activity in cells .
Result of Action
The inhibition of myosin ATPase by this compound can lead to a remarkable increase in the number of domes in epithelial cells, indicating increased transport activity . It also causes a reduction of the transepithelial electrical resistance (TER), leading to an increase in the paracellular flux of small molecular weight dextran .
Biochemical Analysis
Biochemical Properties
2,3-Butanedione-D6 interacts with several enzymes, proteins, and other biomolecules. It is known to be involved in the metabolism of l-lactate by Staphylococcus aureus and Staphylococcus epidermidis . The nature of these interactions is complex and involves various biochemical reactions .
Cellular Effects
The effects of this compound on various types of cells and cellular processes are significant. It influences cell function, including impacts on cell signaling pathways, gene expression, and cellular metabolism . For instance, it has been observed to induce ion and fluid transport in MDCK monolayers .
Molecular Mechanism
At the molecular level, this compound exerts its effects through binding interactions with biomolecules, enzyme inhibition or activation, and changes in gene expression
Temporal Effects in Laboratory Settings
Over time in laboratory settings, the effects of this compound may change. This includes information on the product’s stability, degradation, and any long-term effects on cellular function observed in in vitro or in vivo studies .
Dosage Effects in Animal Models
The effects of this compound vary with different dosages in animal models . For instance, increasing the dose of this compound during cardiopulmonary resuscitation improves resuscitability in a dose-dependent fashion in a pig model of cardiac arrest .
Metabolic Pathways
This compound is involved in several metabolic pathways. It interacts with enzymes or cofactors and can affect metabolic flux or metabolite levels . For example, it has been used in metabolic engineering of non-pathogenic microorganisms for 2,3-butanediol production .
Transport and Distribution
The transport and distribution of this compound within cells and tissues involve various transporters or binding proteins
Subcellular Localization
The subcellular localization of this compound and its effects on activity or function are complex. It could involve targeting signals or post-translational modifications that direct it to specific compartments or organelles
Preparation Methods
Synthetic Routes and Reaction Conditions
The synthesis of 2,3-Butanedione-D6 typically involves the deuteration of 2,3-butanedione. One common method is the exchange of hydrogen atoms with deuterium atoms using deuterium oxide (D2O) as the deuterium source. The reaction is usually carried out under acidic or basic conditions to facilitate the exchange process .
Industrial Production Methods
Industrial production of this compound follows similar principles as laboratory synthesis but on a larger scale. The process involves the use of deuterium gas or deuterium oxide in reactors designed to handle large volumes of chemicals. The reaction conditions are optimized to achieve high yields and purity of the deuterated product .
Chemical Reactions Analysis
Types of Reactions
2,3-Butanedione-D6 undergoes various chemical reactions, including:
Oxidation: It can be oxidized to form acetic acid and carbon dioxide.
Reduction: It can be reduced to form 2,3-butanediol.
Cyclocondensation: It reacts with amines to form triazine and pteridine ring systems.
Common Reagents and Conditions
Oxidation: Common oxidizing agents include potassium permanganate (KMnO4) and chromium trioxide (CrO3).
Reduction: Reducing agents such as sodium borohydride (NaBH4) or lithium aluminum hydride (LiAlH4) are used.
Cyclocondensation: Amines are used as reagents under acidic or basic conditions to facilitate the formation of ring systems.
Major Products Formed
Oxidation: Acetic acid and carbon dioxide.
Reduction: 2,3-butanediol.
Cyclocondensation: Triazine and pteridine derivatives.
Comparison with Similar Compounds
Similar Compounds
2,3-Butanedione (Diacetyl): The non-deuterated form of 2,3-Butanedione-D6, commonly used in the food industry for its buttery flavor.
2,3-Pentanedione: Another diketone with similar chemical properties but a different carbon chain length.
Acetoin: A related compound with a hydroxyl group instead of a second carbonyl group.
Uniqueness
This compound is unique due to the presence of deuterium atoms, which provide distinct advantages in research applications. The deuterium labeling allows for precise tracking in metabolic studies and offers insights into reaction mechanisms that are not possible with non-deuterated compounds .
Properties
IUPAC Name |
1,1,1,4,4,4-hexadeuteriobutane-2,3-dione | |
---|---|---|
Source | PubChem | |
URL | https://pubchem.ncbi.nlm.nih.gov | |
Description | Data deposited in or computed by PubChem | |
InChI |
InChI=1S/C4H6O2/c1-3(5)4(2)6/h1-2H3/i1D3,2D3 | |
Source | PubChem | |
URL | https://pubchem.ncbi.nlm.nih.gov | |
Description | Data deposited in or computed by PubChem | |
InChI Key |
QSJXEFYPDANLFS-WFGJKAKNSA-N | |
Source | PubChem | |
URL | https://pubchem.ncbi.nlm.nih.gov | |
Description | Data deposited in or computed by PubChem | |
Canonical SMILES |
CC(=O)C(=O)C | |
Source | PubChem | |
URL | https://pubchem.ncbi.nlm.nih.gov | |
Description | Data deposited in or computed by PubChem | |
Isomeric SMILES |
[2H]C([2H])([2H])C(=O)C(=O)C([2H])([2H])[2H] | |
Source | PubChem | |
URL | https://pubchem.ncbi.nlm.nih.gov | |
Description | Data deposited in or computed by PubChem | |
Molecular Formula |
C4H6O2 | |
Source | PubChem | |
URL | https://pubchem.ncbi.nlm.nih.gov | |
Description | Data deposited in or computed by PubChem | |
Molecular Weight |
92.13 g/mol | |
Source | PubChem | |
URL | https://pubchem.ncbi.nlm.nih.gov | |
Description | Data deposited in or computed by PubChem | |
Synthesis routes and methods I
Procedure details
Synthesis routes and methods II
Procedure details
Synthesis routes and methods III
Procedure details
Retrosynthesis Analysis
AI-Powered Synthesis Planning: Our tool employs the Template_relevance Pistachio, Template_relevance Bkms_metabolic, Template_relevance Pistachio_ringbreaker, Template_relevance Reaxys, Template_relevance Reaxys_biocatalysis model, leveraging a vast database of chemical reactions to predict feasible synthetic routes.
One-Step Synthesis Focus: Specifically designed for one-step synthesis, it provides concise and direct routes for your target compounds, streamlining the synthesis process.
Accurate Predictions: Utilizing the extensive PISTACHIO, BKMS_METABOLIC, PISTACHIO_RINGBREAKER, REAXYS, REAXYS_BIOCATALYSIS database, our tool offers high-accuracy predictions, reflecting the latest in chemical research and data.
Strategy Settings
Precursor scoring | Relevance Heuristic |
---|---|
Min. plausibility | 0.01 |
Model | Template_relevance |
Template Set | Pistachio/Bkms_metabolic/Pistachio_ringbreaker/Reaxys/Reaxys_biocatalysis |
Top-N result to add to graph | 6 |
Feasible Synthetic Routes
Disclaimer and Information on In-Vitro Research Products
Please be aware that all articles and product information presented on BenchChem are intended solely for informational purposes. The products available for purchase on BenchChem are specifically designed for in-vitro studies, which are conducted outside of living organisms. In-vitro studies, derived from the Latin term "in glass," involve experiments performed in controlled laboratory settings using cells or tissues. It is important to note that these products are not categorized as medicines or drugs, and they have not received approval from the FDA for the prevention, treatment, or cure of any medical condition, ailment, or disease. We must emphasize that any form of bodily introduction of these products into humans or animals is strictly prohibited by law. It is essential to adhere to these guidelines to ensure compliance with legal and ethical standards in research and experimentation.