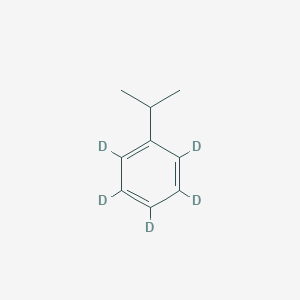
2-Phenyl-D5-propane
Overview
Description
2-Phenyl-D5-propane is a deuterated form of 2-Phenylpropane, which is a colorless liquid with a sweet odor. This compound is commonly used as a solvent and as a starting material for the synthesis of various organic compounds. The deuterated form, this compound, is particularly valuable in research and medical applications due to its unique properties.
Preparation Methods
Synthetic Routes and Reaction Conditions
The synthesis of 2-Phenyl-D5-propane typically involves the deuteration of 2-Phenylpropane. This process can be achieved through catalytic hydrogenation using deuterium gas (D2) in the presence of a suitable catalyst such as palladium on carbon (Pd/C). The reaction is carried out under controlled conditions to ensure the selective incorporation of deuterium atoms into the molecule.
Industrial Production Methods
Industrial production of this compound follows similar synthetic routes but on a larger scale. The process involves the use of high-pressure reactors and advanced catalytic systems to achieve efficient deuteration. The purity and yield of the final product are critical factors in industrial production, and rigorous quality control measures are implemented to ensure consistency .
Chemical Reactions Analysis
Types of Reactions
2-Phenyl-D5-propane undergoes various chemical reactions, including:
Oxidation: The benzylic position (carbon adjacent to the aromatic ring) is particularly reactive and can undergo oxidation to form benzylic alcohols or carboxylic acids.
Reduction: Reduction reactions can convert the compound into different deuterated derivatives.
Substitution: The aromatic ring can participate in electrophilic aromatic substitution reactions, leading to the formation of substituted derivatives.
Common Reagents and Conditions
Oxidation: Common oxidizing agents include potassium permanganate (KMnO4) and chromium trioxide (CrO3).
Reduction: Catalytic hydrogenation using deuterium gas (D2) and palladium on carbon (Pd/C) as a catalyst.
Substitution: Electrophilic aromatic substitution reactions often use reagents such as bromine (Br2) or nitric acid (HNO3) under acidic conditions
Major Products Formed
Scientific Research Applications
2-Phenyl-D5-propane has a wide range of applications in scientific research, including:
Chemistry: Used as a solvent and starting material for the synthesis of various organic compounds.
Biology: Employed in metabolic studies to trace the incorporation and transformation of deuterated compounds in biological systems.
Medicine: Utilized in the development of deuterated drugs, which can exhibit improved pharmacokinetic properties compared to their non-deuterated counterparts.
Industry: Applied in the production of specialty chemicals and materials with enhanced properties.
Mechanism of Action
The mechanism of action of 2-Phenyl-D5-propane involves its interaction with molecular targets and pathways in biological systems. The deuterium atoms in the compound can influence the rate of metabolic reactions, leading to altered pharmacokinetics and dynamics. This property is particularly valuable in drug development, where deuterated compounds can exhibit improved stability and reduced metabolic degradation .
Comparison with Similar Compounds
Similar Compounds
2-Phenylpropane: The non-deuterated form of 2-Phenyl-D5-propane, commonly used as a solvent and starting material.
Phenylpropene: Compounds containing a phenyl ring bonded to propene, used in various chemical syntheses.
Phenylacetone: An organic compound with a similar structure, used in the synthesis of pharmaceuticals and other chemicals.
Uniqueness
This compound is unique due to the presence of deuterium atoms, which confer distinct properties such as altered metabolic pathways and improved stability. These characteristics make it particularly valuable in research and medical applications, where precise tracking and enhanced performance are required.
Properties
IUPAC Name |
1,2,3,4,5-pentadeuterio-6-propan-2-ylbenzene | |
---|---|---|
Source | PubChem | |
URL | https://pubchem.ncbi.nlm.nih.gov | |
Description | Data deposited in or computed by PubChem | |
InChI |
InChI=1S/C9H12/c1-8(2)9-6-4-3-5-7-9/h3-8H,1-2H3/i3D,4D,5D,6D,7D | |
Source | PubChem | |
URL | https://pubchem.ncbi.nlm.nih.gov | |
Description | Data deposited in or computed by PubChem | |
InChI Key |
RWGFKTVRMDUZSP-DKFMXDSJSA-N | |
Source | PubChem | |
URL | https://pubchem.ncbi.nlm.nih.gov | |
Description | Data deposited in or computed by PubChem | |
Canonical SMILES |
CC(C)C1=CC=CC=C1 | |
Source | PubChem | |
URL | https://pubchem.ncbi.nlm.nih.gov | |
Description | Data deposited in or computed by PubChem | |
Isomeric SMILES |
[2H]C1=C(C(=C(C(=C1[2H])[2H])C(C)C)[2H])[2H] | |
Source | PubChem | |
URL | https://pubchem.ncbi.nlm.nih.gov | |
Description | Data deposited in or computed by PubChem | |
Molecular Formula |
C9H12 | |
Source | PubChem | |
URL | https://pubchem.ncbi.nlm.nih.gov | |
Description | Data deposited in or computed by PubChem | |
Molecular Weight |
125.22 g/mol | |
Source | PubChem | |
URL | https://pubchem.ncbi.nlm.nih.gov | |
Description | Data deposited in or computed by PubChem | |
Synthesis routes and methods I
Procedure details
Synthesis routes and methods II
Procedure details
Synthesis routes and methods III
Procedure details
Synthesis routes and methods IV
Procedure details
Synthesis routes and methods V
Procedure details
Retrosynthesis Analysis
AI-Powered Synthesis Planning: Our tool employs the Template_relevance Pistachio, Template_relevance Bkms_metabolic, Template_relevance Pistachio_ringbreaker, Template_relevance Reaxys, Template_relevance Reaxys_biocatalysis model, leveraging a vast database of chemical reactions to predict feasible synthetic routes.
One-Step Synthesis Focus: Specifically designed for one-step synthesis, it provides concise and direct routes for your target compounds, streamlining the synthesis process.
Accurate Predictions: Utilizing the extensive PISTACHIO, BKMS_METABOLIC, PISTACHIO_RINGBREAKER, REAXYS, REAXYS_BIOCATALYSIS database, our tool offers high-accuracy predictions, reflecting the latest in chemical research and data.
Strategy Settings
Precursor scoring | Relevance Heuristic |
---|---|
Min. plausibility | 0.01 |
Model | Template_relevance |
Template Set | Pistachio/Bkms_metabolic/Pistachio_ringbreaker/Reaxys/Reaxys_biocatalysis |
Top-N result to add to graph | 6 |
Feasible Synthetic Routes
Disclaimer and Information on In-Vitro Research Products
Please be aware that all articles and product information presented on BenchChem are intended solely for informational purposes. The products available for purchase on BenchChem are specifically designed for in-vitro studies, which are conducted outside of living organisms. In-vitro studies, derived from the Latin term "in glass," involve experiments performed in controlled laboratory settings using cells or tissues. It is important to note that these products are not categorized as medicines or drugs, and they have not received approval from the FDA for the prevention, treatment, or cure of any medical condition, ailment, or disease. We must emphasize that any form of bodily introduction of these products into humans or animals is strictly prohibited by law. It is essential to adhere to these guidelines to ensure compliance with legal and ethical standards in research and experimentation.