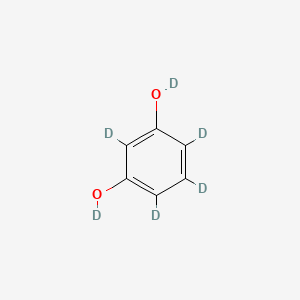
1,3-Dihydroxybenzene-d6
Overview
Description
1,3-Dihydroxybenzene-d6, also known as resorcinol-d6, is a deuterated analogue of resorcinol, which is a phenolic compound widely used in the chemical industry . The “d6” in the name refers to the six deuterium atoms that have replaced six hydrogen atoms in the molecule, making it an isotopically labeled compound . It is mainly used in research and analytical applications, particularly in the field of NMR spectroscopy .
Synthesis Analysis
Resorcinol is produced in several steps from benzene, starting with dialkylation with propylene to give 1,3-diisopropylbenzene . Oxidation and Hock rearrangement of this disubstituted arene gives acetone and resorcinol . A new method for synthesis of Benzene-d6 was discovered, where [Cp*PMe3IrH3] [OTf] was used as the catalyst at H/D exchange reaction between benzene and deuterium oxide .
Molecular Structure Analysis
The molecular formula of 1,3-Dihydroxybenzene-d6 is C6D6O2 . The structure of resorcinol (1,3-dihydroxybenzene) cations has been presented in a study . The adiabatic ionization potentials of the dihydroxybenzenes and the vibrations of the ionic ground state of the dihydroxybenzene cations are experimentally obtained by mass analyzed threshold ionization (MATI) and infrared photoinduced Rydberg ionization (IR-PIRI) spectroscopy .
Chemical Reactions Analysis
The oxidation of resorcinol is a complicated process. At both macro- and micro-electrodes, cyclic voltammetry of resorcinol is chemically and electrochemically irreversible over the whole pH range (1–14). Resorcinol molecules undergo a 1H+ 1e oxidation at pH < pKa1 and a 1e oxidation at pH > pKa2 to form radicals .
Physical And Chemical Properties Analysis
1,3-Dihydroxybenzene-d6 is a colorless crystalline solid with a molecular weight of 116.15 g/mol . It is soluble in water, ethanol, and ether .
Scientific Research Applications
Wastewater Treatment:
1,3-Dihydroxybenzene-d6 is utilized in the electrochemical oxidation process for effective wastewater treatment. This process involves assessing the impact of parameters like the concentration of 1,3-dihydroxybenzene, medium nature (pH), electrolysis time, and organic matter conversion coefficient. A high conversion coefficient of 1,3-dihydroxybenzene, indicating effective treatment, was achieved in a coaxial membraneless electrochemical reactor (Zhelovitskaya, Dresvyannikov, & Shagidullin, 2020).
Electrochemical Synthesis:
The compound plays a role in electrochemical reactions, particularly in the electrochemical synthesis of new o-dihydroxybenzene derivatives. These reactions involve o-quinones derived from o-dihydroxybenzenes participating in 1,4-(Michael) addition reactions with nucleophiles to form corresponding derivatives (Moghaddam et al., 2005).
pKa Determination and Chemical Analysis:
1,3-Dihydroxybenzene-d6 is also significant in the determination of pKa values of hydroxyl groups in its derivatives, which is crucial for understanding their chemical behavior in various processes like advanced oxidation processes (AOPs), oxidative stress, wood biodegradation, and in relation to neuronal diseases in humans. This determination helps in understanding the chelation properties and activities related to the deprotonation of hydroxyl groups (Romero et al., 2018).
Polymerization and Material Science:
In material science, 1,3-dihydroxybenzene-d6 derivatives are used in the curing of polymers, playing a significant role in enhancing the tensile strength and modulus of elastomers through the 1,3-dipolar cycloaddition reaction. This indicates its importance in the development and enhancement of material properties (Fan et al., 2018).
Analytical Chemistry:
The compound's derivatives are integral in analytical chemistry, particularly in the voltammetric analysis of dihydroxybenzene isomers. This involves enhancing electrocatalytic responses and facilitating simultaneous determination without prior separation, essential for applications like wastewater analysis (Ding et al., 2005).
Mechanism of Action
- Role : By interacting with skin cells, resorcinol helps remove hard, scaly, or roughened skin. It also exhibits antibacterial properties, making it effective against conditions like acne, seborrheic dermatitis, eczema, and warts .
- Resorcinol inhibits peroxidases in the thyroid, blocking the synthesis of thyroid hormones and causing goiter. It interferes with iodination of tyrosine and oxidation of iodide. In vitro studies show that resorcinol irreversibly inhibits lactoperoxidase (LPO) and thyroid peroxidase (TPO) through suicide inactivation .
Target of Action
Mode of Action
properties
IUPAC Name |
1,2,3,5-tetradeuterio-4,6-dideuteriooxybenzene | |
---|---|---|
Source | PubChem | |
URL | https://pubchem.ncbi.nlm.nih.gov | |
Description | Data deposited in or computed by PubChem | |
InChI |
InChI=1S/C6H6O2/c7-5-2-1-3-6(8)4-5/h1-4,7-8H/i1D,2D,3D,4D/hD2 | |
Source | PubChem | |
URL | https://pubchem.ncbi.nlm.nih.gov | |
Description | Data deposited in or computed by PubChem | |
InChI Key |
GHMLBKRAJCXXBS-UDDMDDBKSA-N | |
Source | PubChem | |
URL | https://pubchem.ncbi.nlm.nih.gov | |
Description | Data deposited in or computed by PubChem | |
Canonical SMILES |
C1=CC(=CC(=C1)O)O | |
Source | PubChem | |
URL | https://pubchem.ncbi.nlm.nih.gov | |
Description | Data deposited in or computed by PubChem | |
Isomeric SMILES |
[2H]C1=C(C(=C(C(=C1[2H])O[2H])[2H])O[2H])[2H] | |
Source | PubChem | |
URL | https://pubchem.ncbi.nlm.nih.gov | |
Description | Data deposited in or computed by PubChem | |
Molecular Formula |
C6H6O2 | |
Source | PubChem | |
URL | https://pubchem.ncbi.nlm.nih.gov | |
Description | Data deposited in or computed by PubChem | |
DSSTOX Substance ID |
DTXSID80733208 | |
Record name | (~2~H_4_)Benzene-1,3-(~2~H_2_)diol | |
Source | EPA DSSTox | |
URL | https://comptox.epa.gov/dashboard/DTXSID80733208 | |
Description | DSSTox provides a high quality public chemistry resource for supporting improved predictive toxicology. | |
Molecular Weight |
116.15 g/mol | |
Source | PubChem | |
URL | https://pubchem.ncbi.nlm.nih.gov | |
Description | Data deposited in or computed by PubChem | |
Product Name |
1,3-Dihydroxybenzene-d6 | |
CAS RN |
70938-00-0 | |
Record name | (~2~H_4_)Benzene-1,3-(~2~H_2_)diol | |
Source | EPA DSSTox | |
URL | https://comptox.epa.gov/dashboard/DTXSID80733208 | |
Description | DSSTox provides a high quality public chemistry resource for supporting improved predictive toxicology. | |
Record name | 70938-00-0 | |
Source | European Chemicals Agency (ECHA) | |
URL | https://echa.europa.eu/information-on-chemicals | |
Description | The European Chemicals Agency (ECHA) is an agency of the European Union which is the driving force among regulatory authorities in implementing the EU's groundbreaking chemicals legislation for the benefit of human health and the environment as well as for innovation and competitiveness. | |
Explanation | Use of the information, documents and data from the ECHA website is subject to the terms and conditions of this Legal Notice, and subject to other binding limitations provided for under applicable law, the information, documents and data made available on the ECHA website may be reproduced, distributed and/or used, totally or in part, for non-commercial purposes provided that ECHA is acknowledged as the source: "Source: European Chemicals Agency, http://echa.europa.eu/". Such acknowledgement must be included in each copy of the material. ECHA permits and encourages organisations and individuals to create links to the ECHA website under the following cumulative conditions: Links can only be made to webpages that provide a link to the Legal Notice page. | |
Synthesis routes and methods I
Procedure details
Synthesis routes and methods II
Procedure details
Synthesis routes and methods III
Procedure details
Synthesis routes and methods IV
Procedure details
Synthesis routes and methods V
Procedure details
Retrosynthesis Analysis
AI-Powered Synthesis Planning: Our tool employs the Template_relevance Pistachio, Template_relevance Bkms_metabolic, Template_relevance Pistachio_ringbreaker, Template_relevance Reaxys, Template_relevance Reaxys_biocatalysis model, leveraging a vast database of chemical reactions to predict feasible synthetic routes.
One-Step Synthesis Focus: Specifically designed for one-step synthesis, it provides concise and direct routes for your target compounds, streamlining the synthesis process.
Accurate Predictions: Utilizing the extensive PISTACHIO, BKMS_METABOLIC, PISTACHIO_RINGBREAKER, REAXYS, REAXYS_BIOCATALYSIS database, our tool offers high-accuracy predictions, reflecting the latest in chemical research and data.
Strategy Settings
Precursor scoring | Relevance Heuristic |
---|---|
Min. plausibility | 0.01 |
Model | Template_relevance |
Template Set | Pistachio/Bkms_metabolic/Pistachio_ringbreaker/Reaxys/Reaxys_biocatalysis |
Top-N result to add to graph | 6 |
Feasible Synthetic Routes
Disclaimer and Information on In-Vitro Research Products
Please be aware that all articles and product information presented on BenchChem are intended solely for informational purposes. The products available for purchase on BenchChem are specifically designed for in-vitro studies, which are conducted outside of living organisms. In-vitro studies, derived from the Latin term "in glass," involve experiments performed in controlled laboratory settings using cells or tissues. It is important to note that these products are not categorized as medicines or drugs, and they have not received approval from the FDA for the prevention, treatment, or cure of any medical condition, ailment, or disease. We must emphasize that any form of bodily introduction of these products into humans or animals is strictly prohibited by law. It is essential to adhere to these guidelines to ensure compliance with legal and ethical standards in research and experimentation.