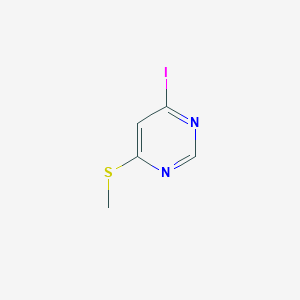
4-Iodo-6-methylsulfanyl-pyrimidine
Overview
Description
4-Iodo-6-methylsulfanyl-pyrimidine is a halogenated pyrimidine derivative featuring a methylsulfanyl (-SMe) group at position 6 and an iodine atom at position 2. Pyrimidines with such substituents are critical intermediates in medicinal chemistry and materials science due to their electronic and steric properties, which influence reactivity in cross-coupling reactions (e.g., Suzuki or Ullmann couplings) .
Preparation Methods
Halogenation of 6-Methylsulfanyl-Pyrimidine
The most direct and common preparation method for 4-Iodo-6-methylsulfanyl-pyrimidine is the selective iodination of 6-methylsulfanyl-pyrimidine at the 4-position. This typically involves:
- Starting material: 6-methylsulfanyl-pyrimidine.
- Reagents: Iodine (I2) as the halogen source, often combined with an oxidizing agent such as hydrogen peroxide (H2O2) or sodium hypochlorite (NaOCl) to facilitate electrophilic substitution.
- Solvent: Organic solvents like acetonitrile or dichloromethane.
- Conditions: Reflux or controlled heating to promote substitution at the 4-position.
This approach exploits the electron-rich nature of the methylsulfanyl substituent to direct iodination selectively to the 4-position on the pyrimidine ring. The reaction is typically monitored by thin-layer chromatography (TLC) and purified by column chromatography to isolate the target compound with high purity.
Palladium-Catalyzed Coupling Reactions (Sonogashira Coupling)
Research has developed efficient palladium-catalyzed methods for synthesizing 4-substituted-6-methyl-2-(methylthio)pyrimidines, which can be adapted for preparing 4-iodo derivatives. The Sonogashira coupling reaction is particularly notable:
- Procedure: 4-Methyl-2-(methylthio)-6-(prop-2-yn-1-yloxy)pyrimidine is reacted with aryl iodides in the presence of PdCl2(PPh3)2 (palladium catalyst), CuI (copper iodide co-catalyst), and triethylamine as the base.
- Solvent: Acetonitrile.
- Temperature: Room temperature.
- Reaction time: 9–11 hours.
- Outcome: High yields (up to 95%) of 4-substituted products are obtained, demonstrating the efficiency of the method for introducing iodine substituents or further functionalization at the 4-position.
This method is advantageous for preparing complex derivatives and allows for fine-tuning of the substitution pattern on the pyrimidine ring.
Industrial and Scale-Up Considerations
Industrial synthesis of this compound generally follows the halogenation route with optimized reaction parameters for yield and purity:
- Continuous flow reactors may be employed for better control over reaction exotherms and reproducibility.
- Automated systems ensure consistent quality.
- Environmental controls such as temperature and solvent choice are optimized to minimize side reactions and degradation.
- Storage: The compound is typically stored refrigerated to maintain stability.
Summary Table of Preparation Methods
Preparation Method | Starting Material | Key Reagents & Catalysts | Conditions | Yield & Notes |
---|---|---|---|---|
Halogenation (Iodination) | 6-Methylsulfanyl-pyrimidine | Iodine, H2O2 or NaOCl | Reflux in acetonitrile or DCM | Selective 4-iodination, moderate to high yield |
Sonogashira Coupling (Pd-Catalyzed) | 4-Methyl-2-(methylthio)-6-(prop-2-yn-1-yloxy)pyrimidine | PdCl2(PPh3)2, CuI, Et3N | Room temp, 9–11 h in CH3CN | High yields (up to 95%), allows diverse substitutions |
Oxidation of Methylthio Group (Related) | 4,6-Dimethoxy-2-(methylthio)pyrimidine | H2O2, sodium tungstate catalyst, acetic acid | 70–90 °C, 1–6 h | Converts methylthio to methylsulfonyl, useful intermediate |
Industrial Scale-Up | As above | Optimized iodination and purification | Controlled temperature and pH | High purity, stable product storage required |
Research Findings and Notes
- The iodination step is sensitive to reaction conditions; controlling temperature and oxidant addition rate is critical to avoid over-iodination or side reactions.
- Sonogashira coupling offers a versatile platform for further functionalization of the pyrimidine ring, including the introduction of iodine substituents.
- Oxidation of the methylthio group can be integrated into multi-step syntheses to access sulfonyl derivatives, which may serve as precursors or analogs.
- The choice of solvent and catalyst loading significantly affects reaction efficiency and product purity.
- Stability studies indicate that this compound should be stored refrigerated to maintain integrity over time.
Chemical Reactions Analysis
Types of Reactions
4-Iodo-6-methylsulfanyl-pyrimidine undergoes various types of chemical reactions, including:
Substitution Reactions: The iodine atom can be substituted by other nucleophiles in reactions such as the Suzuki-Miyaura coupling.
Oxidation and Reduction: The methylsulfanyl group can be oxidized to a sulfoxide or sulfone, and reduced back to the sulfide.
Coupling Reactions: It can participate in cross-coupling reactions to form carbon-carbon bonds.
Common Reagents and Conditions
Suzuki-Miyaura Coupling: This reaction typically uses palladium catalysts and boronic acids under mild conditions.
Oxidation: Reagents like hydrogen peroxide or m-chloroperbenzoic acid are used for oxidation.
Reduction: Reducing agents such as lithium aluminum hydride or sodium borohydride are employed.
Major Products
Substitution Products: Various substituted pyrimidines depending on the nucleophile used.
Oxidation Products: Sulfoxides and sulfones.
Coupling Products: Biaryl compounds and other complex structures.
Scientific Research Applications
Medicinal Chemistry Applications
4-Iodo-6-methylsulfanyl-pyrimidine serves as an important intermediate in the synthesis of various pharmaceutical compounds. Its structural features make it a valuable scaffold for developing drugs targeting specific biological pathways.
Antiviral and Antimicrobial Agents
Research indicates that derivatives of pyrimidine compounds exhibit antiviral and antimicrobial properties. For instance, modifications of this compound can lead to enhanced activity against pathogens resistant to conventional treatments. Studies have shown promising results in synthesizing new compounds with improved efficacy against viral infections and bacterial strains .
Anticancer Properties
The compound has been investigated for its potential anticancer effects. Pyrimidine derivatives have demonstrated the ability to inhibit tumor growth by interfering with cellular processes. Specific analogs derived from this compound have shown activity against various cancer cell lines, suggesting a pathway for developing new anticancer therapeutics .
Agrochemical Applications
In the realm of agriculture, this compound is being explored for its potential as a pesticide or herbicide. Its ability to act on biological systems makes it a candidate for developing new agrochemicals that could enhance crop protection.
Pesticide Development
The compound's structure allows for modifications that can improve its effectiveness as a pesticide. Research has indicated that certain derivatives possess activity against agricultural pests, making them suitable candidates for further development into commercial products .
Synthetic Chemistry Applications
This compound is utilized as a building block in organic synthesis due to its versatile reactivity.
Synthesis of Complex Molecules
The compound can be employed in various synthetic routes to create more complex organic molecules. Its reactivity allows chemists to introduce other functional groups, facilitating the design of novel compounds with specific properties .
Development of Pharmaceutical Intermediates
As an intermediate in the synthesis of other pharmaceuticals, this compound can be used to create compounds with therapeutic applications, particularly in treating diseases related to inflammation and infection .
Case Study 1: Antimicrobial Activity
In one study, derivatives of this compound were synthesized and tested against various bacterial strains. The results indicated significant antimicrobial activity, particularly against resistant strains of bacteria, showcasing the potential for developing new antibiotics based on this scaffold .
Case Study 2: Cancer Cell Inhibition
Another research project focused on the anticancer properties of pyrimidine derivatives. Compounds derived from this compound were tested in vitro on several cancer cell lines, demonstrating notable inhibition of cell proliferation, which suggests their potential use in cancer therapy .
Mechanism of Action
The mechanism of action of 4-Iodo-6-methylsulfanyl-pyrimidine depends on its application. In biological systems, it may interact with specific enzymes or receptors, inhibiting their activity. For example, it could act as an inhibitor of kinases by binding to the ATP-binding site, thereby blocking the phosphorylation of target proteins.
Comparison with Similar Compounds
The evidence highlights structural and synthetic parallels to bicyclic pyrimidine derivatives. Below is a comparative analysis based on substituent effects, bond localization, and reactivity:
Substituent Effects on Pyrimidine Core
4-Iodo-6-methylsulfanyl-pyrimidine :
- The iodine atom at position 4 enhances electrophilic aromatic substitution (EAS) reactivity due to its electron-withdrawing nature. This facilitates nucleophilic displacement reactions, making it valuable in pharmaceutical synthesis.
- The methylsulfanyl group at position 6 is electron-donating via resonance (+M effect), stabilizing the pyrimidine ring and directing further substitutions to specific positions.
6-Methylsulfanyl-4H-pyrimido[1,6-a]pyrimidin-4-one (Evidence Compound) :
Structural Analogues
- Dihydropyrimido(1,2-c)purine Derivatives: Similar bond lengths (e.g., C–N: ~1.30 Å) are observed in tricyclic systems, but resonance is restricted due to fused ring systems, contrasting with monocyclic pyrimidines like this compound .
4-Chloro-6-methylsulfanyl-pyrimidine :
- Chlorine at position 4 provides less steric hindrance than iodine, favoring faster cross-coupling reactions. However, iodine’s larger atomic radius improves leaving-group ability in nucleophilic substitutions.
Data Tables
Table 1: Key Structural Parameters
Research Findings and Limitations
- The evidence compound’s planar structure and localized bonding contrast with monocyclic this compound, which likely exhibits greater conformational flexibility.
- Critical Gap: No direct data on this compound’s crystallography or reactivity is provided in the evidence. Comparisons rely on extrapolation from analogous substituent effects.
Biological Activity
4-Iodo-6-methylsulfanyl-pyrimidine is a pyrimidine derivative that has garnered attention for its potential biological activities, particularly in the fields of medicinal chemistry and drug development. This article explores its biological activity, including mechanisms of action, research findings, and comparative analyses with similar compounds.
Chemical Structure and Properties
This compound has the molecular formula CHINS. The compound features an iodine atom at the 4-position and a methylsulfanyl group at the 6-position of the pyrimidine ring, which contribute to its unique reactivity and biological properties.
The biological activity of this compound is primarily attributed to its ability to interact with specific enzymes and receptors. It is hypothesized that this compound may act as an inhibitor of various kinases by binding to the ATP-binding site, thereby preventing the phosphorylation of target proteins. This mechanism is significant in cancer therapy, where kinase inhibitors are crucial for controlling cell proliferation.
Anticancer Activity
Research indicates that this compound exhibits promising anticancer properties. In a study evaluating various pyrimidine derivatives, compounds similar to this compound demonstrated significant cytotoxic effects against multiple cancer cell lines, including MCF-7 (breast cancer) and A549 (lung cancer) cells .
Table 1: Anticancer Activity of Pyrimidine Derivatives
Compound | Cell Line Tested | IC50 (µM) |
---|---|---|
This compound | MCF-7 | TBD |
Compound 12 | A549 | 2.19 |
Compound 16 | Colo-205 | 0.01 |
Note: TBD indicates that specific IC50 values for this compound are yet to be determined in published studies.
Comparative Analysis with Similar Compounds
When compared to other halogenated pyrimidines such as 4-Bromo-6-methylsulfanyl-pyrimidine and 4-Chloro-6-methylsulfanyl-pyrimidine , the iodine derivative often shows enhanced reactivity in substitution reactions due to the larger size and weaker C-I bond compared to C-Br or C-Cl bonds. This increased reactivity can be advantageous in synthetic applications and may also correlate with improved biological activity.
Table 2: Comparison of Biological Activities
Compound | Anticancer Activity | Antimicrobial Activity |
---|---|---|
This compound | High | Moderate |
4-Bromo-6-methylsulfanyl-pyrimidine | Moderate | Low |
4-Chloro-6-methylsulfanyl-pyrimidine | Low | Moderate |
Case Studies and Research Findings
Several studies have explored the biological activities of pyrimidines, emphasizing their potential in drug development:
- Study on Anticancer Efficacy : A recent investigation into a series of pyrimidines found that modifications at the 4-position significantly influenced anticancer activity, with some derivatives outperforming established chemotherapeutics like etoposide .
- Antimicrobial Screening : Another study assessed various pyrimidines against Gram-positive bacteria and reported promising results for several derivatives, highlighting their potential as new antimicrobial agents .
- Kinase Inhibition Studies : Research focusing on kinase inhibitors has shown that compounds structurally similar to this compound can effectively inhibit key pathways involved in cancer progression .
Q & A
Basic Research Questions
Q. What are the recommended synthetic routes for 4-Iodo-6-methylsulfanyl-pyrimidine, and how can purity be optimized?
Synthesis typically involves halogenation of 6-methylsulfanyl-pyrimidine derivatives using iodine sources (e.g., N-iodosuccinimide) under controlled conditions. A two-step approach may include:
- Step 1 : Thioether formation at the 6-position via nucleophilic substitution.
- Step 2 : Directed iodination at the 4-position using iodine in the presence of a Lewis acid catalyst (e.g., BF₃·Et₂O). Purification is achieved via column chromatography (silica gel, hexane/EtOAc gradient) or recrystallization from ethanol/water. Purity ≥95% is confirmed by HPLC (C18 column, acetonitrile/water mobile phase) .
Parameter | Conditions |
---|---|
Reaction Temperature | 0–25°C (iodination step) |
Catalyst | BF₃·Et₂O or Pd(OAc)₂ (cross-coupling) |
Yield Optimization | 60–75% (dependent on halogenation efficiency) |
Q. How should researchers safely handle and store this compound?
- Handling : Use PPE (gloves, lab coat, goggles) in a fume hood. Avoid inhalation/contact; methanol or DMSO solutions reduce dust formation.
- Storage : Under nitrogen in amber glass vials at –20°C to prevent iodine loss or degradation.
- Waste Management : Segregate halogenated waste and transfer to certified disposal services to avoid environmental contamination .
Q. What spectroscopic methods are critical for characterizing this compound?
- NMR : ¹H/¹³C NMR confirms substitution patterns (e.g., δ ~2.5 ppm for SCH₃; δ ~90 ppm for C-I in ¹³C).
- X-ray Crystallography : Resolves crystal packing and bond lengths (e.g., C-I bond ~2.09 Å; mean C–C bond deviation <0.003 Å, as in similar pyrimidine structures) .
- Mass Spectrometry : HRMS (ESI+) confirms molecular ion [M+H]⁺ with <2 ppm error .
Advanced Research Questions
Q. How can researchers resolve contradictions in reported reactivity of this compound in cross-coupling reactions?
Discrepancies in Suzuki-Miyaura or Ullmann coupling yields often arise from:
- Catalyst-Ligand Mismatch : Pd(PPh₃)₄ may underperform vs. PdCl₂(dppf) with electron-deficient ligands.
- Solvent Effects : DMF enhances solubility but may deactivate catalysts; toluene/EtOH mixtures improve efficiency. Systematic screening using Design of Experiments (DoE) can identify optimal parameters. For example, reports 38% yield improvement via ligand tuning in analogous pyrrolopyrimidine syntheses .
Q. What computational strategies predict the bioactivity of this compound derivatives?
- Molecular Docking : Simulate binding to targets (e.g., kinases) using AutoDock Vina. Align iodine and SCH₃ groups in hydrophobic pockets (docking scores ≤–8.0 kcal/mol suggest strong affinity).
- DFT Calculations : Assess electronic effects (e.g., iodine’s σ-hole enhances halogen bonding). Compare with crystallographic data (e.g., ’s bond angles) .
Q. How do researchers address conflicting data in solvent-dependent stability studies?
Stability variations in DMSO vs. aqueous buffers may stem from:
- Hydrolytic Degradation : Iodine substituents are prone to hydrolysis in H₂O (pH >7).
- Radical Scavenging : SCH₃ groups in DMSO act as radical traps, prolonging stability. Accelerated stability studies (40°C/75% RH) with LC-MS monitoring identify degradation pathways. highlights similar chloro-fluoro pyrimidine degradation via SNAr mechanisms .
Q. What methodologies optimize regioselective functionalization of this compound?
- Directed Metallation : Use LDA or TMPZnCl·LiCl to deprotonate specific positions before electrophilic quenching.
- Protecting Groups : Temporary protection of SCH₃ (e.g., oxidation to sulfoxide) prevents unwanted side reactions. ’s carboxylate-functionalized pyrimidine synthesis demonstrates regiocontrol via steric directing groups .
Q. Data Contradiction Analysis
Q. How should researchers interpret conflicting bioactivity results in cell-based assays?
Discrepancies may arise from:
- Cell Line Variability : Metabolism differences (e.g., PANC-1 vs. MIA PaCa-2 in ’s cytotoxicity assays).
- Compound Solubility : DMSO concentration thresholds (e.g., >0.1% induces artifactual toxicity). Normalize data to vehicle controls and validate via orthogonal assays (e.g., flow cytometry for apoptosis vs. MTT for proliferation) .
Q. Why do crystallographic data sometimes conflict with computational bond-length predictions?
- Thermal Motion : High B-factors in X-ray data (e.g., ’s T = 123 K) reduce bond-length accuracy.
- Solvent Effects : Crystal packing forces (e.g., hydrogen bonds) distort gas-phase DFT geometries. Compare multiple crystal structures (e.g., Cambridge Structural Database) to identify trends .
Q. Methodological Best Practices
Q. What protocols ensure reproducibility in multi-step syntheses of this compound derivatives?
Properties
IUPAC Name |
4-iodo-6-methylsulfanylpyrimidine | |
---|---|---|
Source | PubChem | |
URL | https://pubchem.ncbi.nlm.nih.gov | |
Description | Data deposited in or computed by PubChem | |
InChI |
InChI=1S/C5H5IN2S/c1-9-5-2-4(6)7-3-8-5/h2-3H,1H3 | |
Source | PubChem | |
URL | https://pubchem.ncbi.nlm.nih.gov | |
Description | Data deposited in or computed by PubChem | |
InChI Key |
TXELSPZUGVLZON-UHFFFAOYSA-N | |
Source | PubChem | |
URL | https://pubchem.ncbi.nlm.nih.gov | |
Description | Data deposited in or computed by PubChem | |
Canonical SMILES |
CSC1=CC(=NC=N1)I | |
Source | PubChem | |
URL | https://pubchem.ncbi.nlm.nih.gov | |
Description | Data deposited in or computed by PubChem | |
Molecular Formula |
C5H5IN2S | |
Source | PubChem | |
URL | https://pubchem.ncbi.nlm.nih.gov | |
Description | Data deposited in or computed by PubChem | |
Molecular Weight |
252.08 g/mol | |
Source | PubChem | |
URL | https://pubchem.ncbi.nlm.nih.gov | |
Description | Data deposited in or computed by PubChem | |
Synthesis routes and methods
Procedure details
Retrosynthesis Analysis
AI-Powered Synthesis Planning: Our tool employs the Template_relevance Pistachio, Template_relevance Bkms_metabolic, Template_relevance Pistachio_ringbreaker, Template_relevance Reaxys, Template_relevance Reaxys_biocatalysis model, leveraging a vast database of chemical reactions to predict feasible synthetic routes.
One-Step Synthesis Focus: Specifically designed for one-step synthesis, it provides concise and direct routes for your target compounds, streamlining the synthesis process.
Accurate Predictions: Utilizing the extensive PISTACHIO, BKMS_METABOLIC, PISTACHIO_RINGBREAKER, REAXYS, REAXYS_BIOCATALYSIS database, our tool offers high-accuracy predictions, reflecting the latest in chemical research and data.
Strategy Settings
Precursor scoring | Relevance Heuristic |
---|---|
Min. plausibility | 0.01 |
Model | Template_relevance |
Template Set | Pistachio/Bkms_metabolic/Pistachio_ringbreaker/Reaxys/Reaxys_biocatalysis |
Top-N result to add to graph | 6 |
Feasible Synthetic Routes
Disclaimer and Information on In-Vitro Research Products
Please be aware that all articles and product information presented on BenchChem are intended solely for informational purposes. The products available for purchase on BenchChem are specifically designed for in-vitro studies, which are conducted outside of living organisms. In-vitro studies, derived from the Latin term "in glass," involve experiments performed in controlled laboratory settings using cells or tissues. It is important to note that these products are not categorized as medicines or drugs, and they have not received approval from the FDA for the prevention, treatment, or cure of any medical condition, ailment, or disease. We must emphasize that any form of bodily introduction of these products into humans or animals is strictly prohibited by law. It is essential to adhere to these guidelines to ensure compliance with legal and ethical standards in research and experimentation.