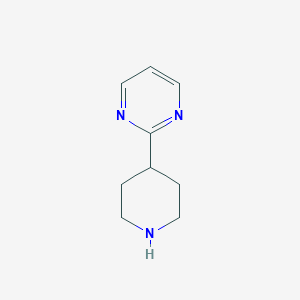
2-(Piperidin-4-YL)pyrimidine
Overview
Description
2-(Piperidin-4-yl)pyrimidine is a heterocyclic compound that features both a piperidine ring and a pyrimidine ring. The piperidine ring is a six-membered ring containing one nitrogen atom, while the pyrimidine ring is a six-membered ring containing two nitrogen atoms at positions 1 and 3. This compound is of significant interest in medicinal chemistry due to its potential pharmacological properties and its role as a building block in the synthesis of various bioactive molecules.
Mechanism of Action
Target of Action
The primary target of 2-(Piperidin-4-YL)pyrimidine is Protein Kinase B (PKB or Akt), a key component of intracellular signaling pathways regulating growth and survival . Piperidine derivatives, including this compound, have been utilized in various therapeutic applications, including anticancer, antiviral, antimalarial, antimicrobial, antifungal, antihypertension, analgesic, anti-inflammatory, anti-Alzheimer, antipsychotic, and/or anticoagulant agents .
Mode of Action
This compound interacts with its target, PKB, in an ATP-competitive manner, providing nano-molar inhibitors with up to 150-fold selectivity for inhibition of PKB over the closely related kinase PKA . This interaction leads to changes in the activity of PKB, affecting its role in cell proliferation and survival .
Biochemical Pathways
The compound’s interaction with PKB affects the phosphatidylinositol-3 kinase (PI3K) signaling pathway . The binding of extracellular growth factors to tyrosine receptor kinases at the cell surface leads to activation of PI3K, which in turn produces phosphatidylinositol-3,4,5 triphosphate (PI(3,4,5)P3) anchored to the inner side of the plasma membrane . Activated PKB signals through phosphorylation of several enzyme or transcription factor substrates, including GSK3β, FKHRL1, BAD, and mTOR, to promote proliferation, protein translation, progression through the cell cycle, and antiapoptotic survival .
Pharmacokinetics
Variation of the linker group between the piperidine and the lipophilic substituent identified 4-amino-1-(7h-pyrrolo[2,3-d]-pyrimidin-4-yl)piperidine-4-carboxamides as potent and orally bioavailable inhibitors of pkb .
Result of Action
The molecular and cellular effects of this compound’s action include modulation of biomarkers of signaling through PKB in vivo and strong inhibition of the growth of human tumor xenografts in nude mice at well-tolerated doses .
Biochemical Analysis
Biochemical Properties
2-(Piperidin-4-YL)pyrimidine has been shown to interact with various enzymes and proteins. For instance, it has been identified as a potent and selective inhibitor of the kinase ERK5 . The nature of these interactions is largely dependent on the specific biochemical context and the other molecules present .
Cellular Effects
The effects of this compound on cells are diverse and complex. It influences cell function by interacting with various cell signaling pathways, affecting gene expression, and altering cellular metabolism .
Molecular Mechanism
At the molecular level, this compound exerts its effects through binding interactions with biomolecules, enzyme inhibition or activation, and changes in gene expression
Preparation Methods
Synthetic Routes and Reaction Conditions
The synthesis of 2-(Piperidin-4-yl)pyrimidine typically involves the reaction of a piperidine derivative with a pyrimidine precursor. One common method is the nucleophilic substitution reaction where a halogenated pyrimidine reacts with piperidine under basic conditions. For example, 2-chloropyrimidine can be reacted with piperidine in the presence of a base such as potassium carbonate to yield this compound.
Industrial Production Methods
In an industrial setting, the production of this compound may involve continuous flow processes to enhance efficiency and yield. Catalysts such as palladium or nickel may be employed to facilitate the reaction, and the use of solvents like dimethylformamide or tetrahydrofuran can improve the solubility of reactants and products.
Chemical Reactions Analysis
Types of Reactions
2-(Piperidin-4-yl)pyrimidine can undergo various chemical reactions, including:
Oxidation: The compound can be oxidized to form N-oxides.
Reduction: Reduction reactions can convert the pyrimidine ring to dihydropyrimidine derivatives.
Substitution: Electrophilic and nucleophilic substitution reactions can introduce different functional groups onto the pyrimidine ring.
Common Reagents and Conditions
Oxidation: Reagents such as hydrogen peroxide or m-chloroperbenzoic acid can be used.
Reduction: Catalytic hydrogenation using palladium on carbon or sodium borohydride.
Substitution: Halogenated derivatives can be used as starting materials, with bases like sodium hydride or potassium tert-butoxide to facilitate the reaction.
Major Products
The major products formed from these reactions include N-oxides, dihydropyrimidine derivatives, and various substituted pyrimidines, depending on the specific reagents and conditions used.
Scientific Research Applications
2-(Piperidin-4-yl)pyrimidine has a wide range of applications in scientific research:
Chemistry: It serves as a building block for the synthesis of more complex heterocyclic compounds.
Biology: The compound is used in the study of enzyme inhibitors and receptor antagonists.
Industry: The compound is used in the production of agrochemicals and other specialty chemicals.
Comparison with Similar Compounds
Similar Compounds
2-(Piperidin-4-yl)pyridine: Similar structure but with a pyridine ring instead of a pyrimidine ring.
2-(Piperidin-4-yl)pyrazine: Contains a pyrazine ring, which has nitrogen atoms at positions 1 and 4.
2-(Piperidin-4-yl)quinoline: Features a quinoline ring, which is a fused ring system with a benzene and pyridine ring.
Uniqueness
2-(Piperidin-4-yl)pyrimidine is unique due to the presence of two nitrogen atoms in the pyrimidine ring, which can participate in hydrogen bonding and other interactions, enhancing its binding affinity and specificity for certain biological targets. This makes it a valuable scaffold in drug design and development.
Properties
IUPAC Name |
2-piperidin-4-ylpyrimidine | |
---|---|---|
Source | PubChem | |
URL | https://pubchem.ncbi.nlm.nih.gov | |
Description | Data deposited in or computed by PubChem | |
InChI |
InChI=1S/C9H13N3/c1-4-11-9(12-5-1)8-2-6-10-7-3-8/h1,4-5,8,10H,2-3,6-7H2 | |
Source | PubChem | |
URL | https://pubchem.ncbi.nlm.nih.gov | |
Description | Data deposited in or computed by PubChem | |
InChI Key |
OLYYSYUUMYTBLJ-UHFFFAOYSA-N | |
Source | PubChem | |
URL | https://pubchem.ncbi.nlm.nih.gov | |
Description | Data deposited in or computed by PubChem | |
Canonical SMILES |
C1CNCCC1C2=NC=CC=N2 | |
Source | PubChem | |
URL | https://pubchem.ncbi.nlm.nih.gov | |
Description | Data deposited in or computed by PubChem | |
Molecular Formula |
C9H13N3 | |
Source | PubChem | |
URL | https://pubchem.ncbi.nlm.nih.gov | |
Description | Data deposited in or computed by PubChem | |
DSSTOX Substance ID |
DTXSID90573551 | |
Record name | 2-(Piperidin-4-yl)pyrimidine | |
Source | EPA DSSTox | |
URL | https://comptox.epa.gov/dashboard/DTXSID90573551 | |
Description | DSSTox provides a high quality public chemistry resource for supporting improved predictive toxicology. | |
Molecular Weight |
163.22 g/mol | |
Source | PubChem | |
URL | https://pubchem.ncbi.nlm.nih.gov | |
Description | Data deposited in or computed by PubChem | |
CAS No. |
151389-25-2 | |
Record name | 2-(Piperidin-4-yl)pyrimidine | |
Source | EPA DSSTox | |
URL | https://comptox.epa.gov/dashboard/DTXSID90573551 | |
Description | DSSTox provides a high quality public chemistry resource for supporting improved predictive toxicology. | |
Synthesis routes and methods I
Procedure details
Synthesis routes and methods II
Procedure details
Retrosynthesis Analysis
AI-Powered Synthesis Planning: Our tool employs the Template_relevance Pistachio, Template_relevance Bkms_metabolic, Template_relevance Pistachio_ringbreaker, Template_relevance Reaxys, Template_relevance Reaxys_biocatalysis model, leveraging a vast database of chemical reactions to predict feasible synthetic routes.
One-Step Synthesis Focus: Specifically designed for one-step synthesis, it provides concise and direct routes for your target compounds, streamlining the synthesis process.
Accurate Predictions: Utilizing the extensive PISTACHIO, BKMS_METABOLIC, PISTACHIO_RINGBREAKER, REAXYS, REAXYS_BIOCATALYSIS database, our tool offers high-accuracy predictions, reflecting the latest in chemical research and data.
Strategy Settings
Precursor scoring | Relevance Heuristic |
---|---|
Min. plausibility | 0.01 |
Model | Template_relevance |
Template Set | Pistachio/Bkms_metabolic/Pistachio_ringbreaker/Reaxys/Reaxys_biocatalysis |
Top-N result to add to graph | 6 |
Feasible Synthetic Routes
Disclaimer and Information on In-Vitro Research Products
Please be aware that all articles and product information presented on BenchChem are intended solely for informational purposes. The products available for purchase on BenchChem are specifically designed for in-vitro studies, which are conducted outside of living organisms. In-vitro studies, derived from the Latin term "in glass," involve experiments performed in controlled laboratory settings using cells or tissues. It is important to note that these products are not categorized as medicines or drugs, and they have not received approval from the FDA for the prevention, treatment, or cure of any medical condition, ailment, or disease. We must emphasize that any form of bodily introduction of these products into humans or animals is strictly prohibited by law. It is essential to adhere to these guidelines to ensure compliance with legal and ethical standards in research and experimentation.