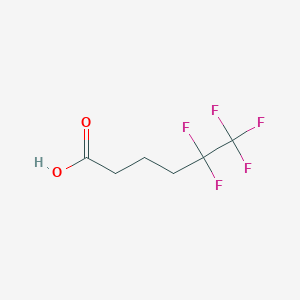
5,5,6,6,6-Pentafluorohexanoic acid
Overview
Description
5,5,6,6,6-Pentafluorohexanoic acid is a fluorinated carboxylic acid with the molecular formula C₆H₇F₅O₂. It is known for its unique chemical properties, including high thermal stability and resistance to degradation. This compound is part of the broader class of per- and polyfluoroalkyl substances (PFAS), which are widely used in various industrial applications due to their hydrophobic and lipophobic characteristics .
Preparation Methods
Synthetic Routes and Reaction Conditions
The synthesis of 5,5,6,6,6-Pentafluorohexanoic acid typically involves the fluorination of hexanoic acid derivatives. One common method is the direct fluorination of hexanoic acid using elemental fluorine under controlled conditions. This process requires careful handling due to the highly reactive nature of fluorine .
Industrial Production Methods
Industrial production of this compound often involves large-scale fluorination processes. These processes are designed to ensure high yield and purity of the final product. The use of specialized reactors and safety protocols is essential to manage the reactivity of fluorine and prevent any hazardous incidents .
Chemical Reactions Analysis
Types of Reactions
5,5,6,6,6-Pentafluorohexanoic acid undergoes various chemical reactions, including:
Oxidation: This compound can be oxidized to form perfluorinated carboxylic acids.
Reduction: Reduction reactions can convert it into less fluorinated derivatives.
Substitution: It can participate in nucleophilic substitution reactions, where the fluorine atoms are replaced by other nucleophiles.
Common Reagents and Conditions
Oxidation: Common oxidizing agents include potassium permanganate and hydrogen peroxide.
Reduction: Reducing agents such as lithium aluminum hydride are used.
Substitution: Nucleophiles like amines and thiols are commonly employed in substitution reactions.
Major Products Formed
The major products formed from these reactions include various fluorinated derivatives, which can be further utilized in different chemical processes and applications .
Scientific Research Applications
5,5,6,6,6-Pentafluorohexanoic acid has a wide range of applications in scientific research:
Chemistry: It is used as a building block for synthesizing more complex fluorinated compounds.
Biology: Its unique properties make it useful in studying the effects of fluorinated compounds on biological systems.
Medicine: Research is ongoing to explore its potential use in drug development and delivery systems.
Mechanism of Action
The mechanism of action of 5,5,6,6,6-Pentafluorohexanoic acid involves its interaction with various molecular targets. Its fluorinated structure allows it to interact with hydrophobic regions of proteins and membranes, potentially altering their function. The exact pathways and molecular targets are still under investigation, but its effects on cellular processes are of significant interest in both biological and chemical research .
Comparison with Similar Compounds
Similar Compounds
Perfluorohexanoic acid: Another fluorinated carboxylic acid with similar properties but a different fluorination pattern.
Perfluorooctanoic acid: A longer-chain fluorinated acid with more extensive industrial use.
Perfluorobutanoic acid: A shorter-chain fluorinated acid with different physical and chemical properties.
Uniqueness
5,5,6,6,6-Pentafluorohexanoic acid is unique due to its specific fluorination pattern, which imparts distinct chemical and physical properties. Its high thermal stability and resistance to degradation make it particularly valuable in applications requiring robust performance under extreme conditions .
Biological Activity
5,5,6,6,6-Pentafluorohexanoic acid (PFHxA) is a perfluoroalkyl acid (PFAA) characterized by its unique fluorinated structure. It has garnered attention due to its presence as a metabolite and degradation product of various fluorotelomer-based substances. Understanding its biological activity is crucial for assessing potential health risks associated with exposure.
- Chemical Formula : C6H7F5O2
- Molecular Weight : 202.12 g/mol
- CAS Number : 12017059
Biological Activity Overview
PFHxA exhibits a range of biological activities and effects on various organisms. Its toxicity and potential health impacts have been the subject of numerous studies, focusing on its carcinogenicity, reproductive toxicity, and endocrine disruption.
Toxicological Profile
- Carcinogenicity : Current research indicates that PFHxA is not carcinogenic. Studies involving Sprague-Dawley rats showed no significant tumor formation associated with PFHxA exposure at various dosage levels .
- Reproductive and Developmental Toxicity : PFHxA does not exhibit selective reproductive or developmental toxicity. Data suggest that it does not disrupt endocrine functions significantly .
- Kidney Effects : The primary concern regarding PFHxA exposure involves potential mild renal effects. Chronic exposure studies indicated histological changes in kidney tissues at high doses, but these effects were generally reversible and occurred at levels much higher than those observed for other perfluorinated compounds like PFOA .
Pharmacokinetics
PFHxA demonstrates rapid absorption and distribution in biological systems:
- Absorption : Following administration, PFHxA is quickly absorbed into the bloodstream.
- Distribution : Studies in rats indicated that PFHxA accumulates in the liver and kidneys, with peak concentrations reached within one hour post-exposure .
- Elimination : The compound undergoes biphasic elimination; rapid elimination occurs initially, followed by a slower phase where lower concentrations persist in the plasma .
Study 1: Human Exposure Assessment
In a study assessing human exposure to PFHxA through drinking water, researchers found detectable levels of PFHxA in various tissues including the brain and liver. The transplacental transfer efficiency was notably high, indicating potential risks for fetal development .
Study 2: Animal Model Research
Research involving repeated oral dosing in rats demonstrated no significant adverse effects on body weight or general health parameters at lower doses. However, higher doses did correlate with decreased survival rates in female subjects .
Data Summary Table
Properties
IUPAC Name |
5,5,6,6,6-pentafluorohexanoic acid | |
---|---|---|
Source | PubChem | |
URL | https://pubchem.ncbi.nlm.nih.gov | |
Description | Data deposited in or computed by PubChem | |
InChI |
InChI=1S/C6H7F5O2/c7-5(8,6(9,10)11)3-1-2-4(12)13/h1-3H2,(H,12,13) | |
Source | PubChem | |
URL | https://pubchem.ncbi.nlm.nih.gov | |
Description | Data deposited in or computed by PubChem | |
InChI Key |
VQNQZVLVFCPHTP-UHFFFAOYSA-N | |
Source | PubChem | |
URL | https://pubchem.ncbi.nlm.nih.gov | |
Description | Data deposited in or computed by PubChem | |
Canonical SMILES |
C(CC(=O)O)CC(C(F)(F)F)(F)F | |
Source | PubChem | |
URL | https://pubchem.ncbi.nlm.nih.gov | |
Description | Data deposited in or computed by PubChem | |
Molecular Formula |
C6H7F5O2 | |
Source | PubChem | |
URL | https://pubchem.ncbi.nlm.nih.gov | |
Description | Data deposited in or computed by PubChem | |
DSSTOX Substance ID |
DTXSID50475885 | |
Record name | 5,5,6,6,6-Pentafluorohexanoic acid | |
Source | EPA DSSTox | |
URL | https://comptox.epa.gov/dashboard/DTXSID50475885 | |
Description | DSSTox provides a high quality public chemistry resource for supporting improved predictive toxicology. | |
Molecular Weight |
206.11 g/mol | |
Source | PubChem | |
URL | https://pubchem.ncbi.nlm.nih.gov | |
Description | Data deposited in or computed by PubChem | |
CAS No. |
148043-70-3 | |
Record name | 5,5,6,6,6-Pentafluorohexanoic acid | |
Source | EPA DSSTox | |
URL | https://comptox.epa.gov/dashboard/DTXSID50475885 | |
Description | DSSTox provides a high quality public chemistry resource for supporting improved predictive toxicology. | |
Synthesis routes and methods I
Procedure details
Synthesis routes and methods II
Procedure details
Retrosynthesis Analysis
AI-Powered Synthesis Planning: Our tool employs the Template_relevance Pistachio, Template_relevance Bkms_metabolic, Template_relevance Pistachio_ringbreaker, Template_relevance Reaxys, Template_relevance Reaxys_biocatalysis model, leveraging a vast database of chemical reactions to predict feasible synthetic routes.
One-Step Synthesis Focus: Specifically designed for one-step synthesis, it provides concise and direct routes for your target compounds, streamlining the synthesis process.
Accurate Predictions: Utilizing the extensive PISTACHIO, BKMS_METABOLIC, PISTACHIO_RINGBREAKER, REAXYS, REAXYS_BIOCATALYSIS database, our tool offers high-accuracy predictions, reflecting the latest in chemical research and data.
Strategy Settings
Precursor scoring | Relevance Heuristic |
---|---|
Min. plausibility | 0.01 |
Model | Template_relevance |
Template Set | Pistachio/Bkms_metabolic/Pistachio_ringbreaker/Reaxys/Reaxys_biocatalysis |
Top-N result to add to graph | 6 |
Feasible Synthetic Routes
Disclaimer and Information on In-Vitro Research Products
Please be aware that all articles and product information presented on BenchChem are intended solely for informational purposes. The products available for purchase on BenchChem are specifically designed for in-vitro studies, which are conducted outside of living organisms. In-vitro studies, derived from the Latin term "in glass," involve experiments performed in controlled laboratory settings using cells or tissues. It is important to note that these products are not categorized as medicines or drugs, and they have not received approval from the FDA for the prevention, treatment, or cure of any medical condition, ailment, or disease. We must emphasize that any form of bodily introduction of these products into humans or animals is strictly prohibited by law. It is essential to adhere to these guidelines to ensure compliance with legal and ethical standards in research and experimentation.