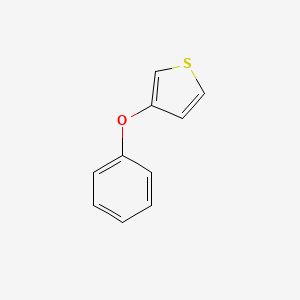
3-Phenoxythiophene
Overview
Description
3-Phenoxythiophene is a chemical compound with the molecular formula C10H8OS and a molecular weight of 176.24 . It is a liquid at room temperature and should be stored in a dark place, sealed in dry conditions .
Synthesis Analysis
The synthesis of thiophene derivatives, such as 3-Phenoxythiophene, often involves ring-forming multicomponent reactions . One method involves the polymerization of 3-hexylthiophene under an electric field . This process improves the regioregularity of the synthesized P3HT .
Molecular Structure Analysis
The molecular structure of 3-Phenoxythiophene can be determined using techniques such as X-ray crystallography . This method allows researchers to provide structures of crystals of a few microns in size .
Chemical Reactions Analysis
3-Phenoxythiophene, like other thiophenes, can undergo a variety of chemical reactions. For instance, it can participate in ring-forming multicomponent reactions to synthesize thiophenes and their derivatives .
Physical And Chemical Properties Analysis
3-Phenoxythiophene has a density of 1.2±0.1 g/cm3 . Its boiling point is 258.0±13.0 °C at 760 mmHg, and it has a vapour pressure of 0.0±0.5 mmHg at 25°C . The compound also has a molar refractivity of 51.1±0.3 cm3 .
Scientific Research Applications
1. Crystal Structure and Self-Assembled Structures
3-Phenoxythiophene and its derivatives, like poly(3-hexyl)thiophene (P3HT), have been studied for their unique crystal structures and self-assembly properties. Research has shown that polyselenophene analogs of P3HT can form a pure type-2 phase, which is stable at room temperature and distinct from P3HT. This finding highlights the potential for these materials to create unique self-assembled structures, motivating further research into selenophene analogs for various applications (Li et al., 2011).
2. Synthesis and Inhibitory Potency
A series of substituted 3-amino-5-phenoxythiophenes has been synthesized, demonstrating significant inhibitory potency for L-T3 uptake in studies on human HepG2 hepatoma cells. The structure of these phenoxythiophenes aligns with general concepts for other classes of L-T3 uptake inhibitors, indicating their potential in medical research (Briel et al., 1999).
3. Reactivity and Electropolymerization
Studies on 3-toluoxythiophene and bithiophenes substituted by toluoxy groups based on the 3-phenoxythiophene building block reveal that the number and position of phenoxy groups significantly influence ground state geometry and reactivity. This impacts their suitability for electropolymerization, indicating potential applications in material science (Leriche et al., 2005).
4. Dye-Sensitized Solar Cells
Phenothiazine derivatives with 3-phenoxythiophene linkers have been used in dye-sensitized solar cells. A study found that derivatives with furan as a conjugated linker showed improved solar energy-to-electricity conversion efficiency, demonstrating the impact of 3-phenoxythiophene in solar cell performance (Kim et al., 2011).
5. Interchain Interactions in Polythiophenes
Research on the absorption spectrum of poly(3-hexylthiophene) (P3HT), a derivative of polythiophene, indicates that interchain interactions must be considered to accurately describe its absorption spectrum. This insight has implications for understanding the physical properties of polythiophenes and their derivatives, such as 3-phenoxythiophene, in various applications (Brown et al., 2003).
6. Electrochromic Polymers
Studies on derivatives of 3,4-ethylenedioxythiophene, which can be related to 3-phenoxythiophene, show potential in creating high-contrast electrochromic polymers. These polymers are interesting for applications where reversible color change and electronic conductivity are desired (Sankaran & Reynolds, 1997).
7. Novel Photochromic Polymers
Azobenzene-substituted polythiophenes, which include 3-phenoxythiophene derivatives, have been synthesized, leading to novel photochromic polymers. These materials offer interesting possibilities in fields requiring materials with photo-responsive properties (Lévesque & Leclerc, 1997).
8. Polymer-Supported Systems for Catalytic Reactions
Poly(3,4-ethylenedioxythiophene) has been used to immobilize metal particle catalysts and reagents for catalytic reactions. This approach, potentially applicable to 3-phenoxythiophene derivatives, demonstrates the utility of polymer-supported systems in catalysis (Sivakumar & Phani, 2011).
9. Conducting Polymers and Electrochemical Devices
Poly(3,4-ethylenedioxythiophene) and its derivatives, including potentially 3-phenoxythiophene, have a broad array of properties making them useful in various electrochemical devices. Their synthetic flexibility and range of optical properties make them suitable for applications like static charge dissipation films and electrode materials (Groenendaal et al., 2000).
Electrochemical Synthesis of Polymers Thiophene and selenophene, when substituted at the 3-position (as in 3-phenoxythiophene), can form polymeric films upon electrochemical oxidation. These polymers, such as poly(3-methylthiophene) and poly(3-methoxythiophene), demonstrate electrical conductivity and undergo reversible color changes, indicating potential applications in electronic and display technologies (Dian, Barbey, & Decroix, 1986).
Photodegradation Applications Polythiophene (PTh) and its derivatives, like 3-phenoxythiophene, show promise in photocatalytic degradation applications. The combination of PTh with metal or metal oxides extends its absorption range and enhances photocatalytic activity under various light conditions. This research opens avenues for environmental applications, particularly in pollution remediation (Ansari et al., 2015).
Organic Solar Cells Dithienosilole-based small molecules incorporating 3-phenoxythiophene units have been designed and synthesized for use in organic solar cells. These molecules, varying slightly in structure, show distinct optical and electrochemical properties, with some achieving power conversion efficiencies over 8%. This highlights the potential of 3-phenoxythiophene derivatives in renewable energy applications (Ni et al., 2015).
Safety and Hazards
3-Phenoxythiophene is toxic if swallowed and can cause skin and eye irritation . It may also cause respiratory irritation . Therefore, it is advised to avoid breathing its dust, fume, gas, mist, vapours, or spray, and to avoid contact with skin and eyes . It should be stored in a well-ventilated place and kept in a locked container .
properties
IUPAC Name |
3-phenoxythiophene | |
---|---|---|
Source | PubChem | |
URL | https://pubchem.ncbi.nlm.nih.gov | |
Description | Data deposited in or computed by PubChem | |
InChI |
InChI=1S/C10H8OS/c1-2-4-9(5-3-1)11-10-6-7-12-8-10/h1-8H | |
Source | PubChem | |
URL | https://pubchem.ncbi.nlm.nih.gov | |
Description | Data deposited in or computed by PubChem | |
InChI Key |
DXSHUWUDKNEGFS-UHFFFAOYSA-N | |
Source | PubChem | |
URL | https://pubchem.ncbi.nlm.nih.gov | |
Description | Data deposited in or computed by PubChem | |
Canonical SMILES |
C1=CC=C(C=C1)OC2=CSC=C2 | |
Source | PubChem | |
URL | https://pubchem.ncbi.nlm.nih.gov | |
Description | Data deposited in or computed by PubChem | |
Molecular Formula |
C10H8OS | |
Source | PubChem | |
URL | https://pubchem.ncbi.nlm.nih.gov | |
Description | Data deposited in or computed by PubChem | |
DSSTOX Substance ID |
DTXSID40427050 | |
Record name | 3-phenoxythiophene | |
Source | EPA DSSTox | |
URL | https://comptox.epa.gov/dashboard/DTXSID40427050 | |
Description | DSSTox provides a high quality public chemistry resource for supporting improved predictive toxicology. | |
Molecular Weight |
176.24 g/mol | |
Source | PubChem | |
URL | https://pubchem.ncbi.nlm.nih.gov | |
Description | Data deposited in or computed by PubChem | |
Product Name |
3-Phenoxythiophene | |
CAS RN |
63285-84-7 | |
Record name | 3-phenoxythiophene | |
Source | EPA DSSTox | |
URL | https://comptox.epa.gov/dashboard/DTXSID40427050 | |
Description | DSSTox provides a high quality public chemistry resource for supporting improved predictive toxicology. | |
Synthesis routes and methods I
Procedure details
Synthesis routes and methods II
Procedure details
Retrosynthesis Analysis
AI-Powered Synthesis Planning: Our tool employs the Template_relevance Pistachio, Template_relevance Bkms_metabolic, Template_relevance Pistachio_ringbreaker, Template_relevance Reaxys, Template_relevance Reaxys_biocatalysis model, leveraging a vast database of chemical reactions to predict feasible synthetic routes.
One-Step Synthesis Focus: Specifically designed for one-step synthesis, it provides concise and direct routes for your target compounds, streamlining the synthesis process.
Accurate Predictions: Utilizing the extensive PISTACHIO, BKMS_METABOLIC, PISTACHIO_RINGBREAKER, REAXYS, REAXYS_BIOCATALYSIS database, our tool offers high-accuracy predictions, reflecting the latest in chemical research and data.
Strategy Settings
Precursor scoring | Relevance Heuristic |
---|---|
Min. plausibility | 0.01 |
Model | Template_relevance |
Template Set | Pistachio/Bkms_metabolic/Pistachio_ringbreaker/Reaxys/Reaxys_biocatalysis |
Top-N result to add to graph | 6 |
Feasible Synthetic Routes
Q & A
Q1: How does the position of the phenoxy group influence the properties of bithiophenes?
A1: [] Both theoretical and experimental studies have demonstrated that the number and position of phenoxy groups significantly impact the geometry of bithiophenes, both in their ground state and as cation radicals. This, in turn, affects the reactivity of these molecules, particularly their ability to undergo electropolymerization. For instance, specific substitutions can lead to more planar structures, facilitating pi-stacking and enhancing conductivity in the resulting polymers.
Q2: What is the significance of using sodium bisulfite in the synthesis of 3-sulphothiophene-2-carboxylic acid?
A2: [] Sodium bisulfite serves as a nucleophile in the copper-mediated nucleophilic substitution reaction used to synthesize 3-sulphothiophene-2-carboxylic acid from the corresponding halothiophenecarboxylic acid. This method is particularly noteworthy because it proposes a novel "ortho halogen assisted intramolecular oxidative addition-reductive elimination mechanism" and offers an improved process for preparing this important pharmaceutical intermediate.
Disclaimer and Information on In-Vitro Research Products
Please be aware that all articles and product information presented on BenchChem are intended solely for informational purposes. The products available for purchase on BenchChem are specifically designed for in-vitro studies, which are conducted outside of living organisms. In-vitro studies, derived from the Latin term "in glass," involve experiments performed in controlled laboratory settings using cells or tissues. It is important to note that these products are not categorized as medicines or drugs, and they have not received approval from the FDA for the prevention, treatment, or cure of any medical condition, ailment, or disease. We must emphasize that any form of bodily introduction of these products into humans or animals is strictly prohibited by law. It is essential to adhere to these guidelines to ensure compliance with legal and ethical standards in research and experimentation.