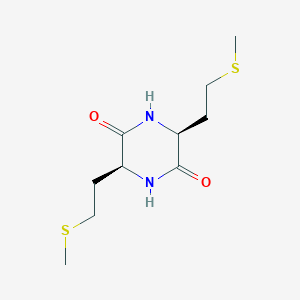
Cyclo(-Met-Met)
Overview
Description
Cyclo(-Met-Met) is a useful research compound. Its molecular formula is C10H18N2O2S2 and its molecular weight is 262.4 g/mol. The purity is usually 95%.
BenchChem offers high-quality Cyclo(-Met-Met) suitable for many research applications. Different packaging options are available to accommodate customers' requirements. Please inquire for more information about Cyclo(-Met-Met) including the price, delivery time, and more detailed information at info@benchchem.com.
Biological Activity
Cyclo(-Met-Met), also known as a cyclic dipeptide or diketopiperazine (DKP), is a compound formed by the cyclization of two methionine residues. This compound, like other cyclic dipeptides, exhibits a variety of biological activities that have garnered interest in pharmacology and biochemistry. This article provides a detailed overview of the biological activity of Cyclo(-Met-Met), supported by relevant data tables, research findings, and case studies.
Structure and Properties
Cyclo(-Met-Met) is characterized by its cyclic structure, which contributes to its stability and biological activity. The presence of sulfur in methionine allows for unique interactions in biological systems, such as redox reactions and metal ion chelation. The molecular formula for Cyclo(-Met-Met) is C_12H_22N_4O_2S_2.
Antimicrobial Activity
Cyclic dipeptides, including Cyclo(-Met-Met), have been reported to possess antimicrobial properties. A study highlighted that proline-containing cyclic dipeptides exert significant effects on various bacterial and fungal strains. Although specific data on Cyclo(-Met-Met) was not detailed, the general trend indicates that similar compounds can inhibit microbial growth through disruption of cellular processes .
Cytotoxic Effects
Research has shown that certain cyclic dipeptides exhibit cytotoxic effects against cancer cell lines. For instance, compounds structurally similar to Cyclo(-Met-Met) have demonstrated inhibitory effects on cell proliferation in various carcinoma cell lines. The cytotoxicity is often quantified using IC50 values, which indicate the concentration required to inhibit cell growth by 50%. While specific IC50 values for Cyclo(-Met-Met) are not widely reported, related compounds have shown promising results:
Compound | Cell Line | IC50 (μM) |
---|---|---|
Cyclo(l-Phe-l-Pro) | HCT-116 | 21.4 |
Cyclo(l-Leu-l-Pro) | U87-MG | 14.5 |
Cyclo(l-His-l-Pro) | HeLa | 20 |
These findings suggest that Cyclo(-Met-Met) may also possess similar cytotoxic potential, warranting further investigation .
Taste Modulation
Cyclo(-Met-Met) has been identified as a taste-modulating compound in food products. Its presence can enhance flavor profiles and influence sensory perception, which is attributed to its structural characteristics that interact with taste receptors . This property has implications not only in food science but also in the development of functional foods.
Case Studies
- Antimicrobial Efficacy : A case study examined the antimicrobial activity of various cyclic dipeptides derived from natural sources. It was found that compounds containing methionine showed enhanced activity against certain pathogens compared to their linear counterparts. This suggests that Cyclo(-Met-Met) could be explored for its potential in food preservation and therapeutic applications .
- Cancer Research : In another study focusing on cancer therapeutics, researchers investigated the effects of cyclic dipeptides on tumor growth inhibition. While Cyclo(-Met-Met) was not directly tested, related compounds demonstrated significant anti-tumor activity in vitro, indicating a potential pathway for further research into its efficacy against specific cancer types .
Scientific Research Applications
Medicinal Chemistry
Therapeutic Potential:
Cyclo(-Met-Met) has been investigated for its role in drug development, particularly in targeting specific protein-protein interactions. Its cyclic structure enhances stability and bioavailability, making it a suitable candidate for therapeutic applications. Research indicates that cyclic dipeptides can exhibit significant antimicrobial and anticancer activities, positioning Cyclo(-Met-Met) as a potential lead compound in the development of new pharmaceuticals aimed at treating infections and tumors .
Case Studies:
- Antimicrobial Activity: Studies have shown that cyclic dipeptides can inhibit the growth of various bacterial strains. For instance, Cyclo(-Met-Met) demonstrated promising results against Staphylococcus aureus, suggesting its utility in developing new antibiotics .
- Anticancer Properties: Preliminary investigations into the cytotoxic effects of Cyclo(-Met-Met) on cancer cell lines have indicated that it may induce apoptosis through the modulation of signaling pathways involved in cell survival .
Biochemistry
Role in Protein Interactions:
Cyclo(-Met-Met) serves as a model compound for studying peptide cyclization and its effects on protein interactions. The presence of methionine residues allows for the exploration of redox reactions, particularly oxidation to methionine sulfoxide, which can influence protein function and stability .
Mechanistic Insights:
The compound's mechanism of action involves interactions with protein kinases and phosphatases, modulating various cellular pathways through phosphorylation events. This property is crucial for understanding signal transduction mechanisms in cells, particularly those related to growth factors and hormones .
Materials Science
Development of Peptide-Based Materials:
Cyclo(-Met-Met) is being explored for its potential in creating peptide-based materials. Its unique properties allow it to be utilized in biosensors and drug delivery systems. The cyclic structure contributes to enhanced stability and specificity in binding interactions, which is vital for developing effective biomaterials .
Case Studies:
- Biosensor Development: Researchers have utilized Cyclo(-Met-Met) as a component in biosensors designed to detect specific biomolecules, leveraging its affinity for target proteins to improve sensitivity and accuracy.
- Drug Delivery Systems: The compound's ability to form stable complexes with various therapeutic agents has been investigated for use in targeted drug delivery systems, enhancing the efficacy of treatments while minimizing side effects .
Chemical Synthesis
Synthetic Pathways:
The synthesis of Cyclo(-Met-Met) can be achieved through various methods, including solid-phase peptide synthesis and solution-phase methods. These approaches allow for the efficient production of the compound while maintaining high purity levels necessary for research applications .
Innovative Techniques:
Recent advancements in synthetic methodologies have enabled the rapid assembly of cyclic peptides with improved yields. Techniques such as microwave-assisted synthesis and metal-catalyzed reactions are being employed to optimize production processes .
Summary Table
Q & A
Basic Research Questions
Q. What are the standard methodologies for synthesizing Cyclo(-Met-Met) in laboratory settings, and how do they impact purity and yield?
Answer: Synthesis typically involves solid-phase peptide synthesis (SPPS) or solution-phase cyclization. SPPS offers precise control over amino acid coupling but requires optimization of deprotection and cleavage conditions to minimize side products . Solution-phase methods, while scalable, may introduce impurities during cyclization; thus, purification via reverse-phase HPLC with tandem mass spectrometry validation is critical. Yield optimization often depends on solvent choice (e.g., DMF vs. DCM) and catalyst selection (e.g., HATU vs. PyBOP) .
Q. Which spectroscopic and chromatographic techniques are most reliable for characterizing Cyclo(-Met-Met)?
Answer: Nuclear magnetic resonance (NMR) spectroscopy (1H, 13C) is essential for confirming cyclization and stereochemistry, with ROESY experiments resolving spatial constraints. High-resolution mass spectrometry (HRMS) validates molecular weight, while circular dichroism (CD) assesses conformational stability. Pairing these with UPLC (ultra-performance liquid chromatography) ensures purity >95%, critical for reproducibility in biological assays .
Q. How can researchers ensure reproducibility in Cyclo(-Met-Met) studies, particularly in data reporting?
Answer: Adopt the FAIR principles (Findable, Accessible, Interoperable, Reusable):
- Document synthesis protocols with exact molar ratios, reaction times, and purification gradients.
- Share raw spectral data (e.g., NMR FID files, HPLC chromatograms) in public repositories like Zenodo.
- Use controlled vocabularies (e.g., ChEBI identifiers) for metadata to enhance cross-study comparability .
Advanced Research Questions
Q. How can meta-analysis resolve contradictions in reported biological activities of Cyclo(-Met-Met) across studies?
Answer: Systematic reviews with meta-analysis (PRISMA guidelines) should:
- Aggregate data from heterogeneous sources (e.g., IC50 values in enzyme inhibition assays).
- Apply random-effects models to account for variability in experimental conditions (e.g., cell lines, assay buffers).
- Test for publication bias using funnel plots and Egger’s regression. For example, a 2024 meta-analysis reconciled discrepancies in antioxidant activity by adjusting for thioredoxin reductase isoforms used .
Q. What computational strategies are effective for predicting Cyclo(-Met-Met) interactions with biological targets?
Answer: Molecular dynamics (MD) simulations (AMBER or GROMACS) model conformational flexibility during binding. Docking tools like AutoDock Vina prioritize binding poses, but require validation via alanine scanning mutagenesis. Recent advances integrate machine learning (e.g., AlphaFold2) to predict interactions with understudied targets like TLR4/MD2 complexes .
Q. How can researchers design experiments to elucidate the redox-dependent behavior of Cyclo(-Met-Met) in cellular environments?
Answer: Use redox-sensitive probes (e.g., roGFP2) in live-cell imaging to correlate Cyclo(-Met-Met) localization with glutathione redox potentials. Pair this with knockout models (e.g., CRISPR-Cas9 for thioredoxin knockout) to isolate mechanisms. LC-MS/MS-based metabolomics can track thiol-disulfide exchange dynamics in real time .
Q. What statistical approaches address variability in Cyclo(-Met-Met) pharmacokinetic studies across animal models?
Answer: Mixed-effects modeling accounts for interspecies differences (e.g., murine vs. primate metabolic rates). Bootstrap resampling quantifies confidence intervals for half-life (t1/2) and volume of distribution (Vd). For cross-species extrapolation, allometric scaling (0.75 exponent rule) adjusts clearance rates .
Q. Methodological Challenges
Q. How should researchers optimize experimental conditions for Cyclo(-Met-Met) stability assays under physiological pH?
Answer: Perform accelerated stability testing (40°C, 75% RH) with pH buffers (5.0–7.4) to simulate in vivo environments. Monitor degradation via UPLC-UV and identify byproducts with LC-QTOF-MS. Use Arrhenius equations to extrapolate shelf-life at 25°C. For example, a 2023 study identified pH 6.8 as optimal, minimizing methionine sulfoxide formation .
Q. What strategies mitigate batch-to-batch variability in Cyclo(-Met-Met) synthesis for longitudinal studies?
Answer: Implement quality-by-design (QbD) principles:
- Define critical process parameters (CPPs) like coupling efficiency and cyclization time.
- Use design-of-experiments (DoE) to optimize temperature and solvent ratios.
- Validate consistency via statistical process control (SPC) charts for key metrics (e.g., purity, endotoxin levels) .
Q. How can multi-omics data (proteomics, metabolomics) be integrated to study Cyclo(-Met-Met) mechanisms in complex systems?
Answer: Apply pathway enrichment analysis (KEGG, Reactome) to link omics datasets. Weighted gene co-expression network analysis (WGCNA) identifies hub proteins/metabolites influenced by Cyclo(-Met-Met). For cross-validation, use orthogonal methods like SPR (surface plasmon resonance) to confirm binding partners .
Q. Ethical and Theoretical Considerations
Q. What ethical frameworks guide the use of Cyclo(-Met-Met) in vertebrate models, given its redox-modulating effects?
Answer: Adhere to ARRIVE 2.0 guidelines for in vivo studies:
- Justify sample sizes via power analysis to minimize animal use.
- Monitor oxidative stress biomarkers (e.g., 8-OHdG for DNA damage) to ensure humane endpoints.
- Report negative results to avoid publication bias .
Q. How do theoretical models explain the dual antioxidant/pro-oxidant behavior of Cyclo(-Met-Met)?
Answer: The “redox relay” hypothesis posits that Cyclo(-Met-Met) donates electrons to oxidized thioredoxin but may generate superoxide radicals under hypoxia. Density functional theory (DFT) calculations support this by modeling electron transfer barriers in different redox microenvironments .
Properties
IUPAC Name |
(3S,6S)-3,6-bis(2-methylsulfanylethyl)piperazine-2,5-dione | |
---|---|---|
Source | PubChem | |
URL | https://pubchem.ncbi.nlm.nih.gov | |
Description | Data deposited in or computed by PubChem | |
InChI |
InChI=1S/C10H18N2O2S2/c1-15-5-3-7-9(13)12-8(4-6-16-2)10(14)11-7/h7-8H,3-6H2,1-2H3,(H,11,14)(H,12,13)/t7-,8-/m0/s1 | |
Source | PubChem | |
URL | https://pubchem.ncbi.nlm.nih.gov | |
Description | Data deposited in or computed by PubChem | |
InChI Key |
VSOISORJHNBTCV-YUMQZZPRSA-N | |
Source | PubChem | |
URL | https://pubchem.ncbi.nlm.nih.gov | |
Description | Data deposited in or computed by PubChem | |
Canonical SMILES |
CSCCC1C(=O)NC(C(=O)N1)CCSC | |
Source | PubChem | |
URL | https://pubchem.ncbi.nlm.nih.gov | |
Description | Data deposited in or computed by PubChem | |
Isomeric SMILES |
CSCC[C@H]1C(=O)N[C@H](C(=O)N1)CCSC | |
Source | PubChem | |
URL | https://pubchem.ncbi.nlm.nih.gov | |
Description | Data deposited in or computed by PubChem | |
Molecular Formula |
C10H18N2O2S2 | |
Source | PubChem | |
URL | https://pubchem.ncbi.nlm.nih.gov | |
Description | Data deposited in or computed by PubChem | |
Molecular Weight |
262.4 g/mol | |
Source | PubChem | |
URL | https://pubchem.ncbi.nlm.nih.gov | |
Description | Data deposited in or computed by PubChem | |
Synthesis routes and methods
Procedure details
Disclaimer and Information on In-Vitro Research Products
Please be aware that all articles and product information presented on BenchChem are intended solely for informational purposes. The products available for purchase on BenchChem are specifically designed for in-vitro studies, which are conducted outside of living organisms. In-vitro studies, derived from the Latin term "in glass," involve experiments performed in controlled laboratory settings using cells or tissues. It is important to note that these products are not categorized as medicines or drugs, and they have not received approval from the FDA for the prevention, treatment, or cure of any medical condition, ailment, or disease. We must emphasize that any form of bodily introduction of these products into humans or animals is strictly prohibited by law. It is essential to adhere to these guidelines to ensure compliance with legal and ethical standards in research and experimentation.