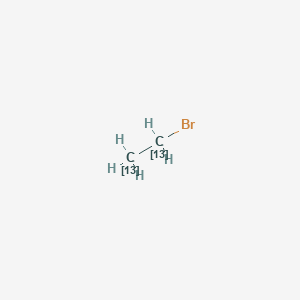
Bromoethane-13C2
Overview
Description
Bromoethane-13C2: is a compound where two carbon atoms in the ethyl group are isotopically labeled with carbon-13. This labeling makes it particularly useful in various scientific research applications, especially in the fields of chemistry and biochemistry. The compound is a clear, colorless liquid with an ethereal odor and a boiling point of 37-40°C .
Preparation Methods
Synthetic Routes and Reaction Conditions: Bromoethane-13C2 can be synthesized through the bromination of ethane-13C2. The reaction typically involves the use of bromine (Br2) in the presence of a catalyst such as iron (Fe) or ultraviolet light to facilitate the substitution reaction. The reaction conditions generally include:
Temperature: Room temperature to slightly elevated temperatures.
Solvent: Often carried out in an inert solvent like carbon tetrachloride (CCl4) to dissolve both reactants.
Catalyst: Iron filings or UV light to initiate the reaction.
Industrial Production Methods: On an industrial scale, the production of this compound follows similar principles but is optimized for larger yields and purity. The process involves:
Continuous Flow Reactors: To maintain consistent reaction conditions and improve efficiency.
Purification Steps: Distillation and recrystallization to achieve high purity levels required for research applications.
Chemical Reactions Analysis
Types of Reactions: Bromoethane-13C2 undergoes several types of chemical reactions, including:
Nucleophilic Substitution (S_N2): This is the most common reaction, where the bromine atom is replaced by a nucleophile. For example, reaction with sodium hydroxide (NaOH) to form ethanol-13C2.
Elimination Reactions (E2): Under strong basic conditions, this compound can undergo elimination to form ethene-13C2.
Oxidation: Although less common, it can be oxidized to form corresponding alcohols or acids under specific conditions.
Common Reagents and Conditions:
Nucleophiles: Sodium hydroxide (NaOH), potassium cyanide (KCN), ammonia (NH3).
Bases: Potassium tert-butoxide (KOtBu) for elimination reactions.
Oxidizing Agents: Potassium permanganate (KMnO4) for oxidation reactions.
Major Products:
Ethanol-13C2: From nucleophilic substitution with hydroxide ions.
Ethene-13C2: From elimination reactions.
Acetic Acid-13C2: From oxidation reactions.
Scientific Research Applications
Chemistry: Bromoethane-13C2 is used as a precursor in the synthesis of various labeled compounds. Its isotopic labeling allows for detailed mechanistic studies using nuclear magnetic resonance (NMR) spectroscopy.
Biology: In biological research, this compound is used to trace metabolic pathways. The carbon-13 label helps in tracking the incorporation and transformation of the compound in biological systems.
Medicine: It is used in the development of labeled pharmaceuticals for diagnostic imaging and metabolic studies. The carbon-13 label provides a non-radioactive alternative for tracing drug metabolism.
Industry: this compound is employed in the production of labeled polymers and materials, aiding in the study of material properties and degradation pathways.
Mechanism of Action
The mechanism by which Bromoethane-13C2 exerts its effects depends on the type of reaction it undergoes. In nucleophilic substitution reactions, the bromine atom is displaced by a nucleophile, forming a new carbon-nucleophile bond. The carbon-13 labeling allows for precise tracking of these reactions using NMR spectroscopy.
Molecular Targets and Pathways:
Nucleophilic Substitution: Targets the carbon-bromine bond, facilitating the formation of new compounds.
Elimination: Involves the removal of a hydrogen atom and a bromine atom, forming a double bond.
Oxidation: Targets the carbon-hydrogen bonds, converting them into carbon-oxygen bonds.
Comparison with Similar Compounds
Iodoethane-13C2: Similar in structure but contains iodine instead of bromine. It is more reactive due to the weaker carbon-iodine bond.
Chloroethane-13C2: Contains chlorine instead of bromine. It is less reactive than Bromoethane-13C2 due to the stronger carbon-chlorine bond.
Fluoroethane-13C2: Contains fluorine. It is the least reactive among the halogenated ethane compounds due to the very strong carbon-fluorine bond.
Uniqueness of this compound: this compound strikes a balance between reactivity and stability, making it a versatile compound for various chemical reactions. Its intermediate reactivity compared to iodoethane-13C2 and chloroethane-13C2 allows for controlled reactions, making it a preferred choice in synthetic chemistry and research applications.
Properties
IUPAC Name |
bromo(1,2-13C2)ethane | |
---|---|---|
Source | PubChem | |
URL | https://pubchem.ncbi.nlm.nih.gov | |
Description | Data deposited in or computed by PubChem | |
InChI |
InChI=1S/C2H5Br/c1-2-3/h2H2,1H3/i1+1,2+1 | |
Source | PubChem | |
URL | https://pubchem.ncbi.nlm.nih.gov | |
Description | Data deposited in or computed by PubChem | |
InChI Key |
RDHPKYGYEGBMSE-ZDOIIHCHSA-N | |
Source | PubChem | |
URL | https://pubchem.ncbi.nlm.nih.gov | |
Description | Data deposited in or computed by PubChem | |
Canonical SMILES |
CCBr | |
Source | PubChem | |
URL | https://pubchem.ncbi.nlm.nih.gov | |
Description | Data deposited in or computed by PubChem | |
Isomeric SMILES |
[13CH3][13CH2]Br | |
Source | PubChem | |
URL | https://pubchem.ncbi.nlm.nih.gov | |
Description | Data deposited in or computed by PubChem | |
Molecular Formula |
C2H5Br | |
Source | PubChem | |
URL | https://pubchem.ncbi.nlm.nih.gov | |
Description | Data deposited in or computed by PubChem | |
DSSTOX Substance ID |
DTXSID30583917 | |
Record name | Bromo(~13~C_2_)ethane | |
Source | EPA DSSTox | |
URL | https://comptox.epa.gov/dashboard/DTXSID30583917 | |
Description | DSSTox provides a high quality public chemistry resource for supporting improved predictive toxicology. | |
Molecular Weight |
110.95 g/mol | |
Source | PubChem | |
URL | https://pubchem.ncbi.nlm.nih.gov | |
Description | Data deposited in or computed by PubChem | |
CAS No. |
34189-75-8 | |
Record name | Bromo(~13~C_2_)ethane | |
Source | EPA DSSTox | |
URL | https://comptox.epa.gov/dashboard/DTXSID30583917 | |
Description | DSSTox provides a high quality public chemistry resource for supporting improved predictive toxicology. | |
Record name | 34189-75-8 | |
Source | European Chemicals Agency (ECHA) | |
URL | https://echa.europa.eu/information-on-chemicals | |
Description | The European Chemicals Agency (ECHA) is an agency of the European Union which is the driving force among regulatory authorities in implementing the EU's groundbreaking chemicals legislation for the benefit of human health and the environment as well as for innovation and competitiveness. | |
Explanation | Use of the information, documents and data from the ECHA website is subject to the terms and conditions of this Legal Notice, and subject to other binding limitations provided for under applicable law, the information, documents and data made available on the ECHA website may be reproduced, distributed and/or used, totally or in part, for non-commercial purposes provided that ECHA is acknowledged as the source: "Source: European Chemicals Agency, http://echa.europa.eu/". Such acknowledgement must be included in each copy of the material. ECHA permits and encourages organisations and individuals to create links to the ECHA website under the following cumulative conditions: Links can only be made to webpages that provide a link to the Legal Notice page. | |
Synthesis routes and methods I
Procedure details
Synthesis routes and methods II
Procedure details
Synthesis routes and methods III
Procedure details
Retrosynthesis Analysis
AI-Powered Synthesis Planning: Our tool employs the Template_relevance Pistachio, Template_relevance Bkms_metabolic, Template_relevance Pistachio_ringbreaker, Template_relevance Reaxys, Template_relevance Reaxys_biocatalysis model, leveraging a vast database of chemical reactions to predict feasible synthetic routes.
One-Step Synthesis Focus: Specifically designed for one-step synthesis, it provides concise and direct routes for your target compounds, streamlining the synthesis process.
Accurate Predictions: Utilizing the extensive PISTACHIO, BKMS_METABOLIC, PISTACHIO_RINGBREAKER, REAXYS, REAXYS_BIOCATALYSIS database, our tool offers high-accuracy predictions, reflecting the latest in chemical research and data.
Strategy Settings
Precursor scoring | Relevance Heuristic |
---|---|
Min. plausibility | 0.01 |
Model | Template_relevance |
Template Set | Pistachio/Bkms_metabolic/Pistachio_ringbreaker/Reaxys/Reaxys_biocatalysis |
Top-N result to add to graph | 6 |
Feasible Synthetic Routes
Disclaimer and Information on In-Vitro Research Products
Please be aware that all articles and product information presented on BenchChem are intended solely for informational purposes. The products available for purchase on BenchChem are specifically designed for in-vitro studies, which are conducted outside of living organisms. In-vitro studies, derived from the Latin term "in glass," involve experiments performed in controlled laboratory settings using cells or tissues. It is important to note that these products are not categorized as medicines or drugs, and they have not received approval from the FDA for the prevention, treatment, or cure of any medical condition, ailment, or disease. We must emphasize that any form of bodily introduction of these products into humans or animals is strictly prohibited by law. It is essential to adhere to these guidelines to ensure compliance with legal and ethical standards in research and experimentation.