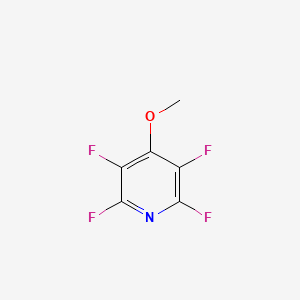
2,3,5,6-Tetrafluoro-4-methoxypyridine
Overview
Description
2,3,5,6-Tetrafluoro-4-methoxypyridine is a fluorinated pyridine derivative with the molecular formula C6H3F4NO. This compound is characterized by the presence of four fluorine atoms and a methoxy group attached to the pyridine ring. It is known for its unique chemical properties, which make it valuable in various scientific and industrial applications.
Preparation Methods
Synthetic Routes and Reaction Conditions: The synthesis of 2,3,5,6-tetrafluoro-4-methoxypyridine typically involves the reaction of phenyl 2,3,5,6-tetrafluoropyridyl sulphone with sodium methoxide. This reaction yields phenyl 2,3,5-trifluoro-6-methoxypyridyl sulphone, which is then treated with caesium fluoride in hot tetramethylene sulphone to produce 2,3,4,5-tetrafluoro-6-methoxypyridine .
Industrial Production Methods: Industrial production methods for this compound are not extensively documented. the synthesis methods mentioned above can be scaled up for industrial applications, ensuring the availability of the compound for various uses.
Chemical Reactions Analysis
Types of Reactions: 2,3,5,6-Tetrafluoro-4-methoxypyridine undergoes several types of chemical reactions, including substitution reactions. For example, hydroxide ion, methoxide ion, and ammonia can attack the compound at the 2-position, leading to the formation of 2-substituted trifluoro-4-iodopyridines .
Common Reagents and Conditions:
Sodium Methoxide: Used in the initial synthesis step.
Caesium Fluoride: Utilized in the subsequent reaction to produce the final compound.
Hydroxide Ion, Methoxide Ion, Ammonia: Employed in substitution reactions.
Major Products Formed: The major products formed from these reactions include various substituted trifluoro-4-iodopyridines, depending on the reagents used.
Scientific Research Applications
2,3,5,6-Tetrafluoro-4-methoxypyridine has several scientific research applications:
Chemistry: Used as a building block in the synthesis of more complex fluorinated compounds.
Medicine: Investigated for its potential use in radiotherapy and as an imaging agent in medical diagnostics.
Industry: Utilized in the production of herbicides and insecticides.
Mechanism of Action
The mechanism of action of 2,3,5,6-tetrafluoro-4-methoxypyridine involves its interaction with various molecular targets and pathways. Under basic conditions, the compound undergoes heterolytic C2-H bond cleavage to form a carbene intermediate, which then eliminates a fluoride ion to produce a cationic species . This cationic species can further react with other molecules, leading to various chemical transformations.
Comparison with Similar Compounds
2,3,5,6-Tetrafluoro-4-trifluoromethylthiopyridine: Prepared by the reaction of pentafluoropyridine with the CF3S- anion.
2,3,5,6-Tetrafluoro-4-iodopyridine: Undergoes substitution reactions similar to 2,3,5,6-tetrafluoro-4-methoxypyridine.
Uniqueness: this compound is unique due to the presence of both fluorine atoms and a methoxy group on the pyridine ring. This combination imparts distinct chemical properties, making it valuable for specific applications in chemistry, biology, and industry.
Properties
IUPAC Name |
2,3,5,6-tetrafluoro-4-methoxypyridine | |
---|---|---|
Source | PubChem | |
URL | https://pubchem.ncbi.nlm.nih.gov | |
Description | Data deposited in or computed by PubChem | |
InChI |
InChI=1S/C6H3F4NO/c1-12-4-2(7)5(9)11-6(10)3(4)8/h1H3 | |
Source | PubChem | |
URL | https://pubchem.ncbi.nlm.nih.gov | |
Description | Data deposited in or computed by PubChem | |
InChI Key |
PBQDQTXTAWOJPP-UHFFFAOYSA-N | |
Source | PubChem | |
URL | https://pubchem.ncbi.nlm.nih.gov | |
Description | Data deposited in or computed by PubChem | |
Canonical SMILES |
COC1=C(C(=NC(=C1F)F)F)F | |
Source | PubChem | |
URL | https://pubchem.ncbi.nlm.nih.gov | |
Description | Data deposited in or computed by PubChem | |
Molecular Formula |
C6H3F4NO | |
Source | PubChem | |
URL | https://pubchem.ncbi.nlm.nih.gov | |
Description | Data deposited in or computed by PubChem | |
DSSTOX Substance ID |
DTXSID50553975 | |
Record name | 2,3,5,6-Tetrafluoro-4-methoxypyridine | |
Source | EPA DSSTox | |
URL | https://comptox.epa.gov/dashboard/DTXSID50553975 | |
Description | DSSTox provides a high quality public chemistry resource for supporting improved predictive toxicology. | |
Molecular Weight |
181.09 g/mol | |
Source | PubChem | |
URL | https://pubchem.ncbi.nlm.nih.gov | |
Description | Data deposited in or computed by PubChem | |
CAS No. |
2375-90-8 | |
Record name | 2,3,5,6-Tetrafluoro-4-methoxypyridine | |
Source | EPA DSSTox | |
URL | https://comptox.epa.gov/dashboard/DTXSID50553975 | |
Description | DSSTox provides a high quality public chemistry resource for supporting improved predictive toxicology. | |
Synthesis routes and methods I
Procedure details
Synthesis routes and methods II
Procedure details
Synthesis routes and methods III
Procedure details
Retrosynthesis Analysis
AI-Powered Synthesis Planning: Our tool employs the Template_relevance Pistachio, Template_relevance Bkms_metabolic, Template_relevance Pistachio_ringbreaker, Template_relevance Reaxys, Template_relevance Reaxys_biocatalysis model, leveraging a vast database of chemical reactions to predict feasible synthetic routes.
One-Step Synthesis Focus: Specifically designed for one-step synthesis, it provides concise and direct routes for your target compounds, streamlining the synthesis process.
Accurate Predictions: Utilizing the extensive PISTACHIO, BKMS_METABOLIC, PISTACHIO_RINGBREAKER, REAXYS, REAXYS_BIOCATALYSIS database, our tool offers high-accuracy predictions, reflecting the latest in chemical research and data.
Strategy Settings
Precursor scoring | Relevance Heuristic |
---|---|
Min. plausibility | 0.01 |
Model | Template_relevance |
Template Set | Pistachio/Bkms_metabolic/Pistachio_ringbreaker/Reaxys/Reaxys_biocatalysis |
Top-N result to add to graph | 6 |
Feasible Synthetic Routes
Q1: What makes 2,3,5,6-Tetrafluoro-4-methoxypyridine particularly reactive in organometallic chemistry?
A1: this compound exhibits unique reactivity with certain transition metal complexes. Specifically, it undergoes C-O oxidative addition with Pt(PCyp3)2 (Cyp = cyclopentyl), leading to the cleavage of the ether O-methyl bond and formation of platinum methyl derivatives. [] This reactivity is unusual, as C-F or C-H activation is typically favored in these types of reactions. [] This preference for C-O bond activation makes this compound a valuable reagent for exploring new synthetic pathways in organometallic chemistry.
Q2: How does this compound compare to other fluorinated pyridines in terms of its reactivity with nucleophiles?
A2: Research indicates that this compound can be selectively modified by nucleophilic substitution. For example, reaction with sodium azide leads to the displacement of the fluorine atom at the 2-position, yielding 2-azido-3,5,6-trifluoro-4-methoxypyridine. [] This highlights the influence of the fluorine substituents on the reactivity of the pyridine ring, enabling selective functionalization for further chemical transformations.
Disclaimer and Information on In-Vitro Research Products
Please be aware that all articles and product information presented on BenchChem are intended solely for informational purposes. The products available for purchase on BenchChem are specifically designed for in-vitro studies, which are conducted outside of living organisms. In-vitro studies, derived from the Latin term "in glass," involve experiments performed in controlled laboratory settings using cells or tissues. It is important to note that these products are not categorized as medicines or drugs, and they have not received approval from the FDA for the prevention, treatment, or cure of any medical condition, ailment, or disease. We must emphasize that any form of bodily introduction of these products into humans or animals is strictly prohibited by law. It is essential to adhere to these guidelines to ensure compliance with legal and ethical standards in research and experimentation.