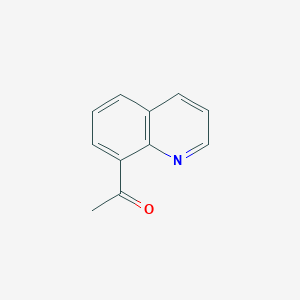
8-Acetylquinoline
Overview
Description
8-Acetylquinoline is a derivative of quinoline, a heterocyclic aromatic organic compound. Quinoline itself is a nitrogen-based compound with a benzene ring fused to a pyridine ring. This compound features an acetyl group (CH₃CO) attached to the eighth position of the quinoline ring. This compound is of significant interest due to its versatile applications in various fields, including medicinal chemistry, organic synthesis, and material science.
Preparation Methods
Synthetic Routes and Reaction Conditions: 8-Acetylquinoline can be synthesized through several methods. One common approach involves the Friedländer synthesis, where aniline derivatives react with α,β-unsaturated aldehydes under acidic or basic conditions. Another method is the Skraup synthesis, which involves the cyclization of aniline with glycerol and sulfuric acid in the presence of an oxidizing agent.
Industrial Production Methods: Industrial production of this compound often employs catalytic systems to enhance yield and efficiency. Transition metal-catalyzed reactions, such as palladium-catalyzed cross-coupling reactions, are frequently used. Additionally, green chemistry approaches, including solvent-free reactions and microwave-assisted synthesis, are gaining popularity due to their environmental benefits .
Chemical Reactions Analysis
Types of Reactions: 8-Acetylquinoline undergoes various chemical reactions, including:
Oxidation: It can be oxidized to form quinoline-8-carboxylic acid.
Reduction: Reduction reactions can convert it into 8-ethylquinoline.
Substitution: Electrophilic and nucleophilic substitution reactions are common, where the acetyl group can be replaced by other functional groups.
Common Reagents and Conditions:
Oxidation: Common oxidizing agents include potassium permanganate and chromium trioxide.
Reduction: Reducing agents like lithium aluminum hydride or sodium borohydride are used.
Substitution: Reagents such as alkyl halides, aryl halides, and various nucleophiles are employed under appropriate conditions.
Major Products:
Oxidation: Quinoline-8-carboxylic acid.
Reduction: 8-Ethylquinoline.
Substitution: Various substituted quinoline derivatives depending on the reagents used
Scientific Research Applications
Medicinal Applications
8-Acetylquinoline and its derivatives exhibit a range of biological activities that make them promising candidates in pharmacology.
Anticancer Activity
Several studies have highlighted the anticancer properties of this compound derivatives. For instance, complexes of 8-hydroxyquinoline with metal ions such as zinc and copper have demonstrated cytotoxic effects against various cancer cell lines, including hepatoma and non-small-cell lung cancer cells. The mechanism involves the chelation of metal ions, which enhances the therapeutic potential of these compounds by inducing apoptosis in cancer cells .
Table 1: Anticancer Activity of this compound Derivatives
Compound Type | Target Cancer Type | IC50 (µM) | Reference |
---|---|---|---|
Zinc Complex | Hepatoma | 5.2 | Chan et al., 2013 |
Copper Complex | Non-small-cell lung cancer | 3.7 | Lam et al., 2016 |
5,7-Dihalo-Substituted | Ovarian cancer | 4.5 | Zhai et al., 2010 |
Antiviral Properties
Recent investigations have also explored the antiviral potential of this compound derivatives against viruses such as dengue. Novel derivatives synthesized showed significant inhibitory activity, with selectivity indices indicating their potential as antiviral agents .
Table 2: Antiviral Activity of this compound Derivatives
Compound | Virus Targeted | IC50 (µM) | CC50 (µM) | Selectivity Index |
---|---|---|---|---|
Iso-Pr Substituted | Dengue Virus | 3.03 | 16.06 | 5.30 |
Iso-Bu Substituted | Dengue Virus | 0.49 | 19.39 | 39.5 |
Neuroprotective Applications
The neuroprotective properties of this compound are attributed to its ability to chelate metal ions such as iron, which are implicated in neurodegenerative diseases like Alzheimer's disease. By reducing metal ion-induced oxidative stress, these compounds can potentially mitigate neuronal damage .
Table 3: Neuroprotective Effects of Metal Chelation
Metal Ion | Effect | Reference |
---|---|---|
Iron | Reduces oxidative stress | Hirbod et al., 2017 |
Zinc | Inhibits amyloid plaque formation | Zhang et al., 2008 |
Material Science Applications
In addition to biological applications, this compound is utilized in material science, particularly in organic electronics.
Organic Light-Emitting Diodes (OLEDs)
The compound serves as an electron transport material in OLEDs due to its favorable electronic properties, enhancing device efficiency and stability .
Table 4: Properties of OLEDs Utilizing this compound
Property | Value |
---|---|
Electron Mobility | High |
Stability | Enhanced |
Case Studies
Several case studies have documented the synthesis and application of various derivatives of this compound.
Case Study: Synthesis of Anticancer Agents
A recent study focused on synthesizing a series of novel derivatives incorporating coumarin and this compound moieties, evaluating their activity against acetylcholinesterase and butyrylcholinesterase enzymes which are relevant for Alzheimer's treatment. These compounds exhibited promising inhibitory activities with IC50 values significantly lower than the standard drug donepezil .
Case Study: Development of Antiviral Compounds
Another case study investigated the synthesis of new derivatives aimed at combating viral infections, specifically targeting dengue virus serotype 2. The study's findings indicated that structural modifications could enhance antiviral efficacy, paving the way for further research into therapeutic applications .
Mechanism of Action
The mechanism of action of 8-Acetylquinoline varies depending on its application. In medicinal chemistry, it often acts by interacting with specific molecular targets, such as enzymes or receptors. For example, it can inhibit the activity of certain enzymes by binding to their active sites, thereby blocking substrate access. In biological systems, it may chelate metal ions, affecting various biochemical pathways .
Comparison with Similar Compounds
Quinoline: The parent compound, used in the synthesis of various derivatives.
8-Hydroxyquinoline: Known for its metal-chelating properties and used in medicinal applications.
8-Methylquinoline: Another derivative with different substituents affecting its chemical behavior.
Uniqueness of 8-Acetylquinoline: this compound is unique due to the presence of the acetyl group, which imparts distinct chemical reactivity and biological activity. This functional group allows for specific interactions in chemical reactions and biological systems, making it a valuable compound in various research fields .
Biological Activity
8-Acetylquinoline, a derivative of quinoline, has garnered significant attention in recent years due to its diverse biological activities. This article delves into its pharmacological potential, including antimicrobial, anticancer, and neuroprotective properties, supported by recent research findings and case studies.
Chemical Structure and Synthesis
This compound (C_10H_9NO) features an acetyl group attached to the nitrogen-containing quinoline ring. The synthesis of this compound typically involves the acetylation of quinoline derivatives, which can be achieved through various methods, including Friedel-Crafts acylation and other catalytic processes. Recent advances in synthetic methodologies have enabled the efficient production of this compound and its derivatives.
1. Antimicrobial Properties
This compound exhibits notable antimicrobial activity against various pathogens. Studies have shown that it can inhibit the growth of bacteria such as Staphylococcus aureus and Klebsiella pneumoniae. For instance, a study reported inhibition zones of 22 mm for Pseudomonas aeruginosa compared to a standard drug with a zone of 24 mm, indicating competitive efficacy .
Pathogen | Inhibition Zone (mm) | Standard Drug Zone (mm) |
---|---|---|
Pseudomonas aeruginosa | 22 | 24 |
Klebsiella pneumoniae | 25 | 27 |
2. Anticancer Activity
The anticancer potential of this compound has been substantiated through various in vitro studies. It acts by inducing oxidative stress and DNA damage in cancer cells, leading to apoptosis. For example, complexes formed with metal ions like Cu(II) have shown IC50 values below 1 µM against several cancer cell lines .
Case Study:
A study investigated the effects of this compound derivatives on HeLa cervical cancer cells. The results indicated that these compounds significantly reduced cell viability through mechanisms involving reactive oxygen species (ROS) generation and cell cycle arrest .
Compound | IC50 (µM) | Mechanism of Action |
---|---|---|
Cu(II) complex with 8-AcQ | <1 | Induction of apoptosis via ROS |
This compound | Varies | DNA damage and cell cycle arrest |
3. Neuroprotective Effects
Research has also highlighted the neuroprotective properties of this compound. It acts as an iron chelator, which is crucial for preventing neurodegenerative diseases linked to metal ion toxicity. Its derivatives have been explored for their potential in treating conditions like Alzheimer's disease by mitigating oxidative stress in neuronal cells .
Structure-Activity Relationships (SAR)
Understanding the structure-activity relationships of this compound is vital for designing more potent derivatives. Modifications at various positions on the quinoline ring can significantly influence biological activity. For example, increasing lipophilicity has been correlated with enhanced antiviral activity against emerging viral pathogens .
Properties
IUPAC Name |
1-quinolin-8-ylethanone | |
---|---|---|
Source | PubChem | |
URL | https://pubchem.ncbi.nlm.nih.gov | |
Description | Data deposited in or computed by PubChem | |
InChI |
InChI=1S/C11H9NO/c1-8(13)10-6-2-4-9-5-3-7-12-11(9)10/h2-7H,1H3 | |
Source | PubChem | |
URL | https://pubchem.ncbi.nlm.nih.gov | |
Description | Data deposited in or computed by PubChem | |
InChI Key |
SHVCKCOMBWIPBE-UHFFFAOYSA-N | |
Source | PubChem | |
URL | https://pubchem.ncbi.nlm.nih.gov | |
Description | Data deposited in or computed by PubChem | |
Canonical SMILES |
CC(=O)C1=CC=CC2=C1N=CC=C2 | |
Source | PubChem | |
URL | https://pubchem.ncbi.nlm.nih.gov | |
Description | Data deposited in or computed by PubChem | |
Molecular Formula |
C11H9NO | |
Source | PubChem | |
URL | https://pubchem.ncbi.nlm.nih.gov | |
Description | Data deposited in or computed by PubChem | |
DSSTOX Substance ID |
DTXSID50480515 | |
Record name | 8-ACETYLQUINOLINE | |
Source | EPA DSSTox | |
URL | https://comptox.epa.gov/dashboard/DTXSID50480515 | |
Description | DSSTox provides a high quality public chemistry resource for supporting improved predictive toxicology. | |
Molecular Weight |
171.19 g/mol | |
Source | PubChem | |
URL | https://pubchem.ncbi.nlm.nih.gov | |
Description | Data deposited in or computed by PubChem | |
CAS No. |
56234-20-9 | |
Record name | 8-ACETYLQUINOLINE | |
Source | EPA DSSTox | |
URL | https://comptox.epa.gov/dashboard/DTXSID50480515 | |
Description | DSSTox provides a high quality public chemistry resource for supporting improved predictive toxicology. | |
Synthesis routes and methods I
Procedure details
Synthesis routes and methods II
Procedure details
Retrosynthesis Analysis
AI-Powered Synthesis Planning: Our tool employs the Template_relevance Pistachio, Template_relevance Bkms_metabolic, Template_relevance Pistachio_ringbreaker, Template_relevance Reaxys, Template_relevance Reaxys_biocatalysis model, leveraging a vast database of chemical reactions to predict feasible synthetic routes.
One-Step Synthesis Focus: Specifically designed for one-step synthesis, it provides concise and direct routes for your target compounds, streamlining the synthesis process.
Accurate Predictions: Utilizing the extensive PISTACHIO, BKMS_METABOLIC, PISTACHIO_RINGBREAKER, REAXYS, REAXYS_BIOCATALYSIS database, our tool offers high-accuracy predictions, reflecting the latest in chemical research and data.
Strategy Settings
Precursor scoring | Relevance Heuristic |
---|---|
Min. plausibility | 0.01 |
Model | Template_relevance |
Template Set | Pistachio/Bkms_metabolic/Pistachio_ringbreaker/Reaxys/Reaxys_biocatalysis |
Top-N result to add to graph | 6 |
Feasible Synthetic Routes
Q1: Can 8-Acetylquinoline undergo photocycloaddition reactions, and if so, what are the products?
A: Yes, this compound can participate in photocycloaddition reactions. For instance, when exposed to UV irradiation in benzene, this compound reacts with 2-Morpholinopropenenitrile. This reaction yields two main products:
* [2+2] Photocycloaddition: rel-(2R,2aR,8bS)-8b-acetyl-2-morpholino-1,2,2a,8b-tetrahydrocyclobuta[h]quinoline-2-carbonitrile. [] * [4+2] Photocycloaddition: rel-(5R,8R,10R)-8-acetyl-10-morpholino-5,8-ethano-5,8-dihydroquinoline-10-carbonitrile. []
Q2: How can the [4+2] photocycloaddition product of this compound and 2-Morpholinopropenenitrile be further modified?
A: The compound rel-(5R,8R,10R)-8-acetyl-10-morpholino-5,8-ethano-5,8-dihydroquinoline-10-carbonitrile, formed via [4+2] photocycloaddition, exhibits interesting reactivity: * Thermal Cleavage: It can be thermally cleaved back into the original reactants, this compound and 2-Morpholinopropenenitrile. This reversible reaction has a determined enthalpy (ΔH ≠) of 130.5 ± 8 kJ mol -1 and entropy (ΔS ≠) of 46 ± 3 J mol -1 K -1. [] * Hydrolysis: Hydrolysis of this compound leads to the formation of the tricyclic diketone, rel-(5R,8R)-8-acetyl-5,8-ethano-5,5-dihydroquinolin-10-one. []
Q3: Can this compound be used as a precursor for synthesizing other substituted quinoline derivatives?
A: Yes, this compound serves as a valuable starting material for synthesizing diverse quinoline derivatives. One example is its use in the synthesis of 8-(1-aminoethyl)quinoline. [] Additionally, a patent describes utilizing this compound in the Willgerodt reaction to synthesize 8-quinolineacetic acid. []
Q4: How does incorporating this compound into a terpyridine-like ligand affect the photophysical properties of its platinum(II) complex?
A: Incorporating this compound into a terpyridine-like ligand, forming 2-(8'-quinolinyl)-1,10-phenanthroline (1), significantly influences the photophysical properties of its platinum(II) complex, [Pt(1)Cl]+, compared to the control complex [Pt(3)Cl]+ (where 3 represents 2-(2'-pyridyl)-1,10-phenanthroline): [] * Absorption: [Pt(1)Cl]+ exhibits higher energy charge-transfer absorption. * Emission: [Pt(1)Cl]+ shows slightly lower energy emission with substantial intra-ligand character. * Excited-state Lifetime: [Pt(1)Cl]+ has a longer excited-state lifetime (310 ns) than [Pt(3)Cl]+ (230 ns) in deoxygenated dichloromethane at room temperature. * Activation Barrier for Quenching: [Pt(1)Cl]+ demonstrates a larger activation barrier for thermally induced quenching compared to [Pt(3)Cl]+.
Disclaimer and Information on In-Vitro Research Products
Please be aware that all articles and product information presented on BenchChem are intended solely for informational purposes. The products available for purchase on BenchChem are specifically designed for in-vitro studies, which are conducted outside of living organisms. In-vitro studies, derived from the Latin term "in glass," involve experiments performed in controlled laboratory settings using cells or tissues. It is important to note that these products are not categorized as medicines or drugs, and they have not received approval from the FDA for the prevention, treatment, or cure of any medical condition, ailment, or disease. We must emphasize that any form of bodily introduction of these products into humans or animals is strictly prohibited by law. It is essential to adhere to these guidelines to ensure compliance with legal and ethical standards in research and experimentation.