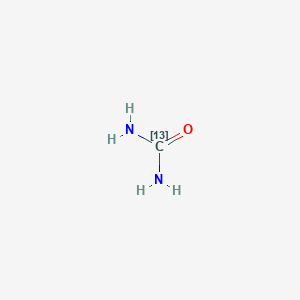
Urea-13C
Overview
Description
Mechanism of Action
Target of Action
The primary target of Urea-13C is the enzyme urease , which is associated with Helicobacter pylori bacteria in the human stomach . This bacterium has been linked to various upper gastrointestinal disorders such as gastritis, gastric and peptic ulcers, stomach cancer, and gastric mucosa-associated lymphoid-tissue (MALT) lymphoma .
Mode of Action
This compound interacts with its target, the urease enzyme, through a process known as hydrolysis . Urease, produced in large quantities by H. pylori, cleaves this compound into ammonia and carbon dioxide . .
Biochemical Pathways
The hydrolysis of this compound by urease affects the carbon metabolism and amino acid biosynthesis pathways . The carbon-13 from this compound is assimilated into these pathways, particularly those important for the production of the hepatotoxin, microcystin . This process allows for the tracing of metabolic pathways within the cell .
Pharmacokinetics
Upon ingestion, if gastric urease from H. pylori is present, this compound is split to form CO2 and NH3 at the interface between the gastric epithelium and lumen . The resulting 13CO2 is absorbed into the blood and exhaled in the breath . Exhaled breath samples can then be collected and measured for the presence of radioactivity . This process forms the basis of the Urea Breath Test (UBT), a diagnostic tool for detecting H. pylori infections .
Result of Action
The action of this compound results in the detection of H. pylori bacteria in the stomach . The presence of these bacteria is associated with various gastrointestinal disorders . Therefore, the action of this compound provides valuable diagnostic information for the initial diagnosis and post-treatment monitoring of H. pylori infection in adults .
Action Environment
The action of this compound can be influenced by environmental factors such as pH conditions . For instance, the incorporation of carbon-13 from this compound into metabolic pathways occurs at a higher percentage during growth at higher pH . This suggests that the action, efficacy, and stability of this compound can be affected by the environmental conditions in which it is used.
Biochemical Analysis
Biochemical Properties
In biochemical reactions, Urea-13C plays a significant role. It interacts with various enzymes, proteins, and other biomolecules. For instance, it can be used in conjunction with dynamic nuclear polarization-enhanced magnetic resonance imaging (MRI) for the study of cancer metabolism .
Cellular Effects
This compound affects various types of cells and cellular processes. It influences cell function, including impacts on cell signaling pathways, gene expression, and cellular metabolism. For example, it can be used to study the metabolic properties of cancer cells, which are vastly different from those of normal cells .
Molecular Mechanism
At the molecular level, this compound exerts its effects through binding interactions with biomolecules, enzyme inhibition or activation, and changes in gene expression. It can be used to trace necrosis, pH changes, and other pathways relevant to cancer .
Metabolic Pathways
This compound is involved in various metabolic pathways. It interacts with enzymes or cofactors and can affect metabolic flux or metabolite levels. For instance, it can be used in 13C metabolic flux analysis (13C-MFA) to determine intracellular fluxes .
Preparation Methods
Synthetic Routes and Reaction Conditions
Urea C-13 is synthesized by incorporating carbon-13 into the urea molecule. The process involves the reaction of carbon-13 labeled carbon dioxide with ammonia to form ammonium carbamate, which is then dehydrated to produce Urea C-13 . The reaction conditions typically involve high pressure and elevated temperatures to facilitate the formation of ammonium carbamate and its subsequent dehydration.
Industrial Production Methods
Industrial production of Urea C-13 follows a similar process to that of regular urea but uses carbon-13 labeled carbon dioxide. The process involves the following steps:
Reaction of Carbon-13 Labeled Carbon Dioxide with Ammonia: This step forms ammonium carbamate.
Dehydration of Ammonium Carbamate: The ammonium carbamate is then dehydrated to produce Urea C-13.
Chemical Reactions Analysis
Types of Reactions
Urea C-13 undergoes various chemical reactions, including:
Hydrolysis: Urea C-13 can be hydrolyzed to produce ammonia and carbon dioxide.
Oxidation: It can undergo oxidation reactions to form different nitrogen oxides.
Decomposition: Urea C-13 decomposes at elevated temperatures to produce ammonia and isocyanic acid.
Common Reagents and Conditions
Hydrolysis: Typically involves water and can be catalyzed by acids or bases.
Oxidation: Common oxidizing agents include potassium permanganate and hydrogen peroxide.
Decomposition: Elevated temperatures are required for the decomposition of Urea C-13.
Major Products Formed
Hydrolysis: Ammonia and carbon dioxide.
Oxidation: Nitrogen oxides.
Decomposition: Ammonia and isocyanic acid.
Scientific Research Applications
Urea C-13 has a wide range of applications in scientific research, including:
Chemistry: Used as a tracer in various chemical reactions to study reaction mechanisms and pathways.
Biology: Employed in metabolic studies to trace the incorporation of nitrogen into biological molecules.
Medicine: Widely used in the urea breath test for detecting Helicobacter pylori infections.
Industry: Utilized in the production of labeled compounds for research and diagnostic purposes
Comparison with Similar Compounds
Similar Compounds
Urea: The non-labeled version of Urea C-13, commonly used in fertilizers and industrial applications.
Thiourea: Similar in structure to urea but contains sulfur instead of oxygen.
Hydroxyurea: Contains a hydroxyl group and is used as a medication for certain types of cancer and sickle cell anemia.
Uniqueness of Urea C-13
Urea C-13 is unique due to its isotopic labeling, which allows it to be used as a tracer in various scientific and medical applications. The carbon-13 isotope provides a distinct signal that can be detected using specialized analytical techniques, making it invaluable for studying metabolic pathways, reaction mechanisms, and diagnosing infections .
Properties
IUPAC Name |
diamino(113C)methanone | |
---|---|---|
Source | PubChem | |
URL | https://pubchem.ncbi.nlm.nih.gov | |
Description | Data deposited in or computed by PubChem | |
InChI |
InChI=1S/CH4N2O/c2-1(3)4/h(H4,2,3,4)/i1+1 | |
Source | PubChem | |
URL | https://pubchem.ncbi.nlm.nih.gov | |
Description | Data deposited in or computed by PubChem | |
InChI Key |
XSQUKJJJFZCRTK-OUBTZVSYSA-N | |
Source | PubChem | |
URL | https://pubchem.ncbi.nlm.nih.gov | |
Description | Data deposited in or computed by PubChem | |
Canonical SMILES |
C(=O)(N)N | |
Source | PubChem | |
URL | https://pubchem.ncbi.nlm.nih.gov | |
Description | Data deposited in or computed by PubChem | |
Isomeric SMILES |
[13C](=O)(N)N | |
Source | PubChem | |
URL | https://pubchem.ncbi.nlm.nih.gov | |
Description | Data deposited in or computed by PubChem | |
Molecular Formula |
CH4N2O | |
Source | PubChem | |
URL | https://pubchem.ncbi.nlm.nih.gov | |
Description | Data deposited in or computed by PubChem | |
DSSTOX Substance ID |
DTXSID20973641 | |
Record name | (~13~C)Carbamimidic acid | |
Source | EPA DSSTox | |
URL | https://comptox.epa.gov/dashboard/DTXSID20973641 | |
Description | DSSTox provides a high quality public chemistry resource for supporting improved predictive toxicology. | |
Molecular Weight |
61.048 g/mol | |
Source | PubChem | |
URL | https://pubchem.ncbi.nlm.nih.gov | |
Description | Data deposited in or computed by PubChem | |
Mechanism of Action |
The Urea Breath Test is based on the ability of the H. pylori enzyme urease to cleave urea into ammonia and carbon dioxide. As the urease enzyme is not present in mammalian cells, the presence of urease (and the products of urea cleavage) in the stomach is evidence that H. pylori bacteria are present. To detect H. pylori, urea labeled with 13C is swallowed by the patient. If gastric urease from H. pylori is present, urea is split to form CO2 and NH3 at the interface between the gastric epithelium and lumen and 13CO2 is absorbed into the blood and exhaled in the breath. Exhaled breath samples can then be collected and measured for the presence of radioactivity. | |
Record name | Urea C-13 | |
Source | DrugBank | |
URL | https://www.drugbank.ca/drugs/DB09510 | |
Description | The DrugBank database is a unique bioinformatics and cheminformatics resource that combines detailed drug (i.e. chemical, pharmacological and pharmaceutical) data with comprehensive drug target (i.e. sequence, structure, and pathway) information. | |
Explanation | Creative Common's Attribution-NonCommercial 4.0 International License (http://creativecommons.org/licenses/by-nc/4.0/legalcode) | |
CAS No. |
58069-82-2 | |
Record name | Urea C 13 [USP] | |
Source | ChemIDplus | |
URL | https://pubchem.ncbi.nlm.nih.gov/substance/?source=chemidplus&sourceid=0058069822 | |
Description | ChemIDplus is a free, web search system that provides access to the structure and nomenclature authority files used for the identification of chemical substances cited in National Library of Medicine (NLM) databases, including the TOXNET system. | |
Record name | Urea C-13 | |
Source | DrugBank | |
URL | https://www.drugbank.ca/drugs/DB09510 | |
Description | The DrugBank database is a unique bioinformatics and cheminformatics resource that combines detailed drug (i.e. chemical, pharmacological and pharmaceutical) data with comprehensive drug target (i.e. sequence, structure, and pathway) information. | |
Explanation | Creative Common's Attribution-NonCommercial 4.0 International License (http://creativecommons.org/licenses/by-nc/4.0/legalcode) | |
Record name | (~13~C)Carbamimidic acid | |
Source | EPA DSSTox | |
URL | https://comptox.epa.gov/dashboard/DTXSID20973641 | |
Description | DSSTox provides a high quality public chemistry resource for supporting improved predictive toxicology. | |
Record name | UREA C-13 | |
Source | FDA Global Substance Registration System (GSRS) | |
URL | https://gsrs.ncats.nih.gov/ginas/app/beta/substances/W6KX9E6D8X | |
Description | The FDA Global Substance Registration System (GSRS) enables the efficient and accurate exchange of information on what substances are in regulated products. Instead of relying on names, which vary across regulatory domains, countries, and regions, the GSRS knowledge base makes it possible for substances to be defined by standardized, scientific descriptions. | |
Explanation | Unless otherwise noted, the contents of the FDA website (www.fda.gov), both text and graphics, are not copyrighted. They are in the public domain and may be republished, reprinted and otherwise used freely by anyone without the need to obtain permission from FDA. Credit to the U.S. Food and Drug Administration as the source is appreciated but not required. | |
Synthesis routes and methods I
Procedure details
Synthesis routes and methods II
Procedure details
Synthesis routes and methods III
Procedure details
Synthesis routes and methods IV
Procedure details
Synthesis routes and methods V
Procedure details
Retrosynthesis Analysis
AI-Powered Synthesis Planning: Our tool employs the Template_relevance Pistachio, Template_relevance Bkms_metabolic, Template_relevance Pistachio_ringbreaker, Template_relevance Reaxys, Template_relevance Reaxys_biocatalysis model, leveraging a vast database of chemical reactions to predict feasible synthetic routes.
One-Step Synthesis Focus: Specifically designed for one-step synthesis, it provides concise and direct routes for your target compounds, streamlining the synthesis process.
Accurate Predictions: Utilizing the extensive PISTACHIO, BKMS_METABOLIC, PISTACHIO_RINGBREAKER, REAXYS, REAXYS_BIOCATALYSIS database, our tool offers high-accuracy predictions, reflecting the latest in chemical research and data.
Strategy Settings
Precursor scoring | Relevance Heuristic |
---|---|
Min. plausibility | 0.01 |
Model | Template_relevance |
Template Set | Pistachio/Bkms_metabolic/Pistachio_ringbreaker/Reaxys/Reaxys_biocatalysis |
Top-N result to add to graph | 6 |
Feasible Synthetic Routes
Q1: How does Urea-13C help diagnose Helicobacter pylori infection?
A1: this compound leverages the unique ability of Helicobacter pylori to produce the enzyme urease. When administered orally, this compound is hydrolyzed by bacterial urease in the stomach, producing ammonia and 13C-labeled carbon dioxide (13CO2). [, ] This 13CO2 is absorbed into the bloodstream and subsequently exhaled. By measuring the 13CO2 enrichment in breath samples using techniques like isotope ratio mass spectrometry, the presence of Helicobacter pylori can be determined. [, , ]
Q2: What is the molecular formula and weight of this compound?
A2: this compound, with a single 13C substitution, has a molecular formula of CH313CONH2 and a molecular weight of 61.06 g/mol.
Q3: Are there any spectroscopic differences between urea and this compound?
A3: Yes, the presence of the 13C isotope in this compound alters its vibrational frequencies, leading to characteristic shifts in its infrared spectrum compared to naturally abundant urea. []
Q4: Is this compound stable under standard storage conditions?
A4: this compound is chemically stable under standard storage conditions.
Q5: Does this compound possess any catalytic properties?
A5: No, this compound does not exhibit inherent catalytic properties. Its utility lies in its role as a substrate for bacterial urease, enabling the detection of Helicobacter pylori.
Q6: Have there been computational studies on the interaction between this compound and urease?
A6: While specific computational studies focusing on this compound might be limited, extensive research exists on the interaction between urea and urease. These studies provide valuable insights into the enzyme's catalytic mechanism and substrate binding. []
Q7: Does modifying the urea structure in this compound affect its diagnostic utility?
A7: Modifying the urea structure in this compound could potentially affect its interaction with urease, impacting its hydrolysis and subsequent diagnostic accuracy. []
Q8: What are the common formulations of this compound for diagnostic use?
A8: this compound is typically formulated in capsules or solutions for oral administration. The formulation is designed to ensure accurate dosing and reliable release of the compound in the stomach. [, ]
Q9: What is the fate of this compound after ingestion?
A9: After oral administration, this compound is rapidly absorbed and distributed throughout the body. If Helicobacter pylori is present, this compound is hydrolyzed in the stomach. The generated 13CO2 is absorbed into the bloodstream and subsequently eliminated through exhalation. Unabsorbed this compound is excreted in the urine. [, ]
Q10: How does the performance of this compound breath test compare to other diagnostic methods for Helicobacter pylori?
A10: The this compound breath test demonstrates high sensitivity and specificity for detecting Helicobacter pylori infection, comparable to invasive methods like biopsy and culture. [, , ] It's considered a reliable non-invasive alternative, particularly in children. [, ]
Q11: Is there any evidence of resistance to the this compound breath test?
A11: Resistance to the this compound breath test is not a concern, as the test principle relies on the presence of the bacterial enzyme urease, a stable characteristic of Helicobacter pylori. [, ]
Q12: How is the 13CO2 enrichment in breath samples measured?
A12: Isotope ratio mass spectrometry (IRMS) is the gold standard for measuring the 13CO2 enrichment in breath samples. [, , ] This technique allows for precise measurement of isotopic ratios, enabling accurate diagnosis.
Q13: What are the key parameters considered during the validation of analytical methods for this compound analysis?
A13: Validation of analytical methods for this compound analysis focuses on parameters like accuracy, precision, specificity, linearity, range, limit of detection, and limit of quantification to ensure reliable and reproducible results. []
Q14: What are some essential resources for research involving this compound?
A14: Essential resources include access to isotopically labeled this compound, specialized equipment like isotope ratio mass spectrometers, and expertise in analytical techniques for isotope analysis. []
Q15: When was this compound first introduced as a diagnostic tool for Helicobacter pylori?
A15: The this compound breath test was introduced in the early 1990s as a non-invasive alternative to traditional methods for detecting Helicobacter pylori infection. []
Disclaimer and Information on In-Vitro Research Products
Please be aware that all articles and product information presented on BenchChem are intended solely for informational purposes. The products available for purchase on BenchChem are specifically designed for in-vitro studies, which are conducted outside of living organisms. In-vitro studies, derived from the Latin term "in glass," involve experiments performed in controlled laboratory settings using cells or tissues. It is important to note that these products are not categorized as medicines or drugs, and they have not received approval from the FDA for the prevention, treatment, or cure of any medical condition, ailment, or disease. We must emphasize that any form of bodily introduction of these products into humans or animals is strictly prohibited by law. It is essential to adhere to these guidelines to ensure compliance with legal and ethical standards in research and experimentation.