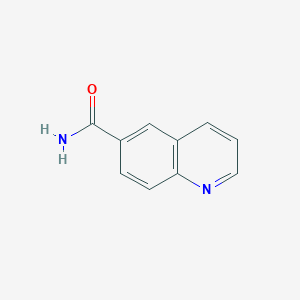
Quinoline-6-carboxamide
Overview
Description
Quinoline-6-carboxamide is a heterocyclic compound featuring a quinoline backbone with a carboxamide group at the 6-position. This structural motif is pivotal in medicinal chemistry due to its versatility in interacting with biological targets, such as DNA topoisomerases and kinase enzymes. The compound is synthesized via palladium-catalyzed aminocarbonylation, which enables high chemoselectivity under optimized conditions (e.g., Pd(OAc)₂/XantPhos catalyst, atmospheric CO pressure, 50°C), yielding derivatives in 13–90% efficiency . Key applications include cytotoxic agents, DNA intercalators, and CB2 receptor ligands, with notable efficacy in tumor models and enzyme inhibition .
Preparation Methods
Synthetic Routes and Reaction Conditions
The synthesis of quinoline-6-carboxamide can be achieved through various methods. One common approach involves the reaction of quinoline-6-carboxylic acid with ammonia or amines under suitable conditions. For instance, the reaction of quinoline-6-carboxylic acid with ammonia in the presence of a dehydrating agent like thionyl chloride can yield this compound .
Industrial Production Methods
Industrial production methods for this compound often involve scalable and efficient synthetic routes. These methods may include the use of microwave-assisted synthesis, solvent-free conditions, and green chemistry approaches to minimize environmental impact .
Chemical Reactions Analysis
Types of Reactions
Quinoline-6-carboxamide undergoes various chemical reactions, including:
Oxidation: It can be oxidized to form quinoline-6-carboxylic acid.
Reduction: Reduction reactions can convert it to quinoline-6-carboxylate.
Substitution: It can undergo substitution reactions, particularly at the nitrogen atom of the carboxamide group.
Common Reagents and Conditions
Oxidation: Common oxidizing agents include potassium permanganate and chromium trioxide.
Reduction: Reducing agents such as lithium aluminum hydride or sodium borohydride are often used.
Substitution: Substitution reactions may involve reagents like alkyl halides or acyl chlorides.
Major Products Formed
The major products formed from these reactions include quinoline-6-carboxylic acid, quinoline-6-carboxylate, and various substituted quinoline derivatives .
Scientific Research Applications
Synthesis of Quinoline-6-Carboxamide Derivatives
The synthesis of this compound derivatives has been extensively studied using various methodologies, particularly palladium-catalyzed reactions. The aminocarbonylation of 6-iodoquinoline has emerged as a prominent method for producing these derivatives. This reaction involves the introduction of amide functionalities into the quinoline structure, which is crucial for enhancing biological activity.
Key Synthetic Routes:
- Palladium-Catalyzed Aminocarbonylation : This method utilizes amine nucleophiles under elevated carbon monoxide pressure to yield quinoline-6-carboxamides with high selectivity and efficiency .
- Buchwald–Hartwig Amination : This approach has been used to functionalize the quinoline ring at position 6, leading to various derivatives that exhibit promising biological properties .
Biological Evaluation and Pharmacological Activities
This compound derivatives have been investigated for their pharmacological activities, particularly as potential therapeutics in treating neurological disorders and cancer.
Pharmacological Properties:
- mGluR1 Antagonists : Some derivatives have shown significant inhibitory activity against the metabotropic glutamate receptor 1 (mGluR1), which is implicated in neuropathic pain. For example, compound 13c demonstrated an IC50 value of 2.16 µM in inhibiting mGluR1, indicating its potential as a lead compound for further development .
- Anti-Cancer Activity : Certain quinoline derivatives have exhibited cytotoxic effects against various cancer cell lines, making them candidates for anticancer drug development. The structure–activity relationship studies suggest that modifications at the 6-position can enhance their efficacy .
Case Studies and Experimental Findings
Several case studies highlight the effectiveness of this compound derivatives in biological systems:
Case Study 1: Neuropathic Pain Model
In a study involving a spinal nerve ligation model for neuropathic pain, compound 13c was administered to rats. The results indicated a reduction in mechanical allodynia and cold allodynia, although its efficacy was not superior to gabapentin, a standard treatment . This study underscores the need for further optimization of quinoline derivatives to enhance their therapeutic potential.
Case Study 2: Antimicrobial Activity
This compound derivatives have also been evaluated for antimicrobial properties. Some compounds demonstrated significant inhibition against bacterial strains, suggesting their utility as antimicrobial agents. The mechanism of action is believed to involve interference with bacterial DNA gyrase activity .
Structure–Activity Relationship (SAR)
Understanding the structure–activity relationship is crucial for optimizing the pharmacological properties of this compound derivatives. Key findings from SAR studies include:
- Substituent Effects : The presence of hydrophobic cyclic amines at the R1 position enhances mGluR1 antagonistic activity. Smaller secondary amide groups at R2 and R3 also contribute positively to inhibitory potency .
- Diversity in Functional Groups : A wide range of amine nucleophiles can be utilized in the synthesis process, allowing for the exploration of diverse chemical space and identification of potent bioactive compounds .
Data Table: Inhibitory Activity of Selected Quinoline Derivatives
Compound | R1 (Amine) | % Inhibition (mGluR1) at 10 µM |
---|---|---|
12b | Pyrrolidine | 65.97 |
13c | Piperidine | 76.76 |
14a | Piperazine | 28.73 |
This table summarizes some key findings related to the inhibitory activities of selected quinoline derivatives against mGluR1, highlighting their potential as therapeutic agents.
Mechanism of Action
The mechanism of action of quinoline-6-carboxamide involves its interaction with specific molecular targets. It has been shown to inhibit protein kinases, which are crucial regulators of cell survival and proliferation. By inhibiting these kinases, this compound can induce apoptosis (programmed cell death) in cancer cells. The compound also affects various signaling pathways involved in cell growth and differentiation .
Comparison with Similar Compounds
Structural Variations and Substituent Effects
The biological and physicochemical properties of quinoline-6-carboxamide derivatives are highly dependent on substituents. Below is a comparison of structurally related compounds:
Table 1: Structural Derivatives and Physical Properties
Key Observations :
- Bulky substituents (e.g., 3-phenylpropyl in compound 17) correlate with higher melting points and improved cytotoxicity .
- Fluorescence intensity in benzimidazo[1,2-a]quinoline derivatives is reduced by ~2-fold when replacing a cyano group (compound 5) with carboxamide (compound 7), indicating electronic effects of substituents on photophysical properties .
Key Insights :
- Indeno[1,2-b]this compound demonstrates efficacy comparable to clinical trial candidate DACA, highlighting the impact of fused ring systems on cytotoxicity .
- 7-Oxo-oxazinothis compound derivatives show enhanced CB2 receptor selectivity, emphasizing the role of heteroatom incorporation in receptor binding .
Critical Analysis :
- The Pd/XantPhos system achieves superior chemoselectivity (>98% carboxamide yield) compared to monodentate ligands like triphenylphosphine .
- Friedländer synthesis is preferred for generating fused-ring systems (e.g., indenoquinolines) but requires optimization for scalability .
Biological Activity
Quinoline-6-carboxamide is a compound that has garnered attention in medicinal chemistry due to its diverse biological activities. This article explores the synthesis, biological evaluations, and potential therapeutic applications of this compound derivatives, highlighting their anticancer, antimicrobial, and anti-inflammatory properties.
1. Synthesis of this compound Derivatives
The synthesis of this compound derivatives typically involves the aminocarbonylation of 6-iodoquinoline with various amine nucleophiles. This method has been optimized to yield high selectivity and efficiency in producing both carboxamides and ketoamides. The reaction conditions, such as carbon monoxide pressure and the choice of catalysts (e.g., Pd(OAc)2 with XantPhos), significantly influence the yield and purity of the products .
Table 1: Summary of Synthesis Conditions for this compound Derivatives
Amine Used | CO Pressure (bar) | Reaction Time (h) | Yield (%) |
---|---|---|---|
Piperidine | 40 | 6 | 75 |
Tert-butylamine | Atmospheric | 2 | 84 |
Cyclohexylamine | Atmospheric | 4 | 90 |
2. Anticancer Activity
Recent studies have demonstrated that this compound derivatives exhibit significant anticancer properties. For example, compounds synthesized from this scaffold have been tested against various cancer cell lines, including breast cancer (MCF-7, SKBR3) and leukemia (RPMI-8226).
One study reported that specific derivatives showed IC50 values as low as 8.50 μM against MCF-7 cells, indicating potent antiproliferative effects. The mechanism of action appears to involve the induction of apoptosis through the activation of caspase pathways and cell cycle arrest at the G2-M phase .
Table 2: Anticancer Activity of Selected this compound Derivatives
Compound | Cell Line | IC50 (μM) | Mechanism |
---|---|---|---|
Compound 5k | MCF-7 | 8.50 | Apoptosis induction, G2-M arrest |
Compound 5l | MDA-MB-231 | 12.51 | Apoptosis induction |
Compound 13c | RPMI-8226 | 0.45 - 0.91 | Caspase-3 activation |
3. Antimicrobial Activity
Quinoline derivatives also exhibit antimicrobial properties. For instance, studies have shown that certain quinoline-based compounds possess activity against multidrug-resistant strains of bacteria and fungi. The structure-activity relationship (SAR) studies indicate that modifications at various positions on the quinoline ring can enhance or diminish antimicrobial efficacy.
For example, a derivative with a chlorine atom at position 6 demonstrated significant activity against Leishmania species with an IC50 value of approximately 0.22 μM, showcasing its potential as an antileishmanial agent .
4. Anti-inflammatory Properties
In addition to their anticancer and antimicrobial activities, this compound derivatives have been investigated for their anti-inflammatory effects. Research indicates that these compounds can modulate inflammatory pathways by inhibiting pro-inflammatory cytokines and enzymes involved in inflammation .
Case Studies
Several case studies illustrate the therapeutic potential of this compound derivatives:
- Breast Cancer Treatment : A study involving a series of synthesized quinoline derivatives showed promising results in inhibiting breast cancer cell proliferation while sparing normal cells, indicating a favorable therapeutic index.
- Leishmaniasis Management : Another study highlighted the efficacy of a specific quinoline derivative in reducing parasite load in infected mice models, suggesting its potential as a treatment for leishmaniasis.
- Tuberculosis : Research on quinoline derivatives has also pointed towards their effectiveness against Mycobacterium tuberculosis, with some compounds exhibiting MIC values as low as 0.5 μg/mL .
Q & A
Basic Research Questions
Q. What are the common synthetic routes for preparing quinoline-6-carboxamide derivatives, and how are they optimized for yield and purity?
this compound derivatives are typically synthesized via palladium-catalyzed aminocarbonylation of 6-iodoquinoline, which allows selective formation of carboxamides or glyoxylamides depending on ligand choice (e.g., XantPhos for carboxamides under atmospheric CO pressure) . Reaction optimization involves adjusting CO pressure, catalyst systems (e.g., Pd(OAc)₂ with monodentate/bidentate ligands), and amine nucleophiles. Purification is achieved via silica gel chromatography with solvent gradients (e.g., CHCl₃:MeOH 50:1 to 20:1) . Yield improvements (up to 98%) rely on ligand selection and solvent ratios .
Q. How are this compound derivatives characterized structurally, and what analytical techniques are critical for validation?
Key characterization methods include:
- Nuclear Magnetic Resonance (NMR) : ¹H and ¹³C NMR identify substituent positions and confirm backbone integrity (e.g., aromatic protons at δ 8.5–9.0 ppm) .
- High-Resolution Mass Spectrometry (HRMS) : Validates molecular weight (e.g., HRMS (ESI) m/z 389.1600 for compound 13 ) .
- Melting Point Analysis : Ensures purity (e.g., 209.2–210.1°C for compound 13 ) .
Q. What biological activities are associated with this compound derivatives, and how are these evaluated in preliminary studies?
Derivatives like Galunisertib (LY2157299) inhibit TGF-β/Smad signaling and are tested in cancer models using cell viability assays (e.g., IC₅₀ values in μM ranges) . Other compounds, such as indolizinoquinolinediones, are screened for DNA topoisomerase inhibition via enzymatic assays and DNA cleavage studies .
Advanced Research Questions
Q. How do reaction conditions influence regioselectivity in this compound synthesis, and how can contradictory data be resolved?
Contradictions in product distribution (e.g., carboxamide vs. glyoxylamide formation) arise from ligand steric/electronic effects. For example, XantPhos promotes carboxamide formation (98% yield) by stabilizing Pd intermediates, whereas PPh₃ favors glyoxylamides (63% yield) under high CO pressure . Discrepancies in reported yields may stem from solvent polarity, temperature, or amine nucleophile reactivity. Resolution requires controlled replication studies with in-situ monitoring (e.g., FTIR for CO uptake) .
Q. What computational methods are used to predict and validate the corrosion inhibition properties of this compound derivatives on metals like mild steel?
Density Functional Theory (DFT) calculates electronic parameters (e.g., HOMO-LUMO energy gaps, Fukui indices) to correlate molecular structure with inhibition efficiency. For example, N-[4-(1,3-benzo[d]thiazol-2-ylcarbamoyl)phenyl]this compound shows strong adsorption on XC38 steel via thiazole and quinoline moieties, validated by electrochemical impedance spectroscopy (EIS) and potentiodynamic polarization . Molecular dynamics simulations model inhibitor-metal surface interactions under acidic conditions .
Q. How do structural modifications (e.g., substituent groups) affect the biochemical efficacy of this compound derivatives in targeting epigenetic regulators?
Substituents like pyrrolidine or morpholine rings modulate target binding. For instance, N-(3-morpholinopropyl)-indolizinoquinolinedione (19 ) exhibits enhanced DNA topoisomerase inhibition due to improved solubility and hydrogen-bonding capacity . In epigenetics, derivatives like N-(2-aminophenyl)-quinoline-6-carboxamide (114a ) target reader domains (e.g., BET proteins) by mimicking acetyl-lysine interactions, validated via X-ray crystallography and fluorescence polarization assays .
Q. What strategies are employed to resolve discrepancies in biological activity data across this compound analogs?
Discrepancies arise from assay conditions (e.g., cell line variability, enzyme isoform specificity). For example, Galunisertib’s TGF-β inhibition varies between epithelial vs. mesenchymal cancer models due to differential receptor expression . Meta-analysis of dose-response curves and orthogonal assays (e.g., Western blotting for Smad2/3 phosphorylation) can clarify mechanisms .
Q. Methodological Guidance
Q. How should researchers design experiments to compare the bioactivity of this compound derivatives with structurally similar compounds?
- Step 1 : Perform SAR (Structure-Activity Relationship) studies by systematically varying substituents (e.g., alkyl chains, heterocycles) .
- Step 2 : Use standardized assays (e.g., MIC for antimicrobial activity, IC₅₀ for enzyme inhibition) with positive/negative controls .
- Step 3 : Apply statistical tools (e.g., ANOVA, PCA) to identify significant structural contributors to activity .
Q. What protocols are recommended for optimizing synthetic scalability of this compound derivatives while maintaining purity?
Properties
IUPAC Name |
quinoline-6-carboxamide | |
---|---|---|
Source | PubChem | |
URL | https://pubchem.ncbi.nlm.nih.gov | |
Description | Data deposited in or computed by PubChem | |
InChI |
InChI=1S/C10H8N2O/c11-10(13)8-3-4-9-7(6-8)2-1-5-12-9/h1-6H,(H2,11,13) | |
Source | PubChem | |
URL | https://pubchem.ncbi.nlm.nih.gov | |
Description | Data deposited in or computed by PubChem | |
InChI Key |
YMNAJWHTELQUJU-UHFFFAOYSA-N | |
Source | PubChem | |
URL | https://pubchem.ncbi.nlm.nih.gov | |
Description | Data deposited in or computed by PubChem | |
Canonical SMILES |
C1=CC2=C(C=CC(=C2)C(=O)N)N=C1 | |
Source | PubChem | |
URL | https://pubchem.ncbi.nlm.nih.gov | |
Description | Data deposited in or computed by PubChem | |
Molecular Formula |
C10H8N2O | |
Source | PubChem | |
URL | https://pubchem.ncbi.nlm.nih.gov | |
Description | Data deposited in or computed by PubChem | |
DSSTOX Substance ID |
DTXSID40453374 | |
Record name | Quinoline-6-carboxamide | |
Source | EPA DSSTox | |
URL | https://comptox.epa.gov/dashboard/DTXSID40453374 | |
Description | DSSTox provides a high quality public chemistry resource for supporting improved predictive toxicology. | |
Molecular Weight |
172.18 g/mol | |
Source | PubChem | |
URL | https://pubchem.ncbi.nlm.nih.gov | |
Description | Data deposited in or computed by PubChem | |
CAS No. |
5382-43-4 | |
Record name | 6-Quinolinecarboxamide | |
Source | CAS Common Chemistry | |
URL | https://commonchemistry.cas.org/detail?cas_rn=5382-43-4 | |
Description | CAS Common Chemistry is an open community resource for accessing chemical information. Nearly 500,000 chemical substances from CAS REGISTRY cover areas of community interest, including common and frequently regulated chemicals, and those relevant to high school and undergraduate chemistry classes. This chemical information, curated by our expert scientists, is provided in alignment with our mission as a division of the American Chemical Society. | |
Explanation | The data from CAS Common Chemistry is provided under a CC-BY-NC 4.0 license, unless otherwise stated. | |
Record name | Quinoline-6-carboxamide | |
Source | EPA DSSTox | |
URL | https://comptox.epa.gov/dashboard/DTXSID40453374 | |
Description | DSSTox provides a high quality public chemistry resource for supporting improved predictive toxicology. | |
Record name | quinoline-6-carboxamide | |
Source | European Chemicals Agency (ECHA) | |
URL | https://echa.europa.eu/information-on-chemicals | |
Description | The European Chemicals Agency (ECHA) is an agency of the European Union which is the driving force among regulatory authorities in implementing the EU's groundbreaking chemicals legislation for the benefit of human health and the environment as well as for innovation and competitiveness. | |
Explanation | Use of the information, documents and data from the ECHA website is subject to the terms and conditions of this Legal Notice, and subject to other binding limitations provided for under applicable law, the information, documents and data made available on the ECHA website may be reproduced, distributed and/or used, totally or in part, for non-commercial purposes provided that ECHA is acknowledged as the source: "Source: European Chemicals Agency, http://echa.europa.eu/". Such acknowledgement must be included in each copy of the material. ECHA permits and encourages organisations and individuals to create links to the ECHA website under the following cumulative conditions: Links can only be made to webpages that provide a link to the Legal Notice page. | |
Synthesis routes and methods I
Procedure details
Synthesis routes and methods II
Procedure details
Retrosynthesis Analysis
AI-Powered Synthesis Planning: Our tool employs the Template_relevance Pistachio, Template_relevance Bkms_metabolic, Template_relevance Pistachio_ringbreaker, Template_relevance Reaxys, Template_relevance Reaxys_biocatalysis model, leveraging a vast database of chemical reactions to predict feasible synthetic routes.
One-Step Synthesis Focus: Specifically designed for one-step synthesis, it provides concise and direct routes for your target compounds, streamlining the synthesis process.
Accurate Predictions: Utilizing the extensive PISTACHIO, BKMS_METABOLIC, PISTACHIO_RINGBREAKER, REAXYS, REAXYS_BIOCATALYSIS database, our tool offers high-accuracy predictions, reflecting the latest in chemical research and data.
Strategy Settings
Precursor scoring | Relevance Heuristic |
---|---|
Min. plausibility | 0.01 |
Model | Template_relevance |
Template Set | Pistachio/Bkms_metabolic/Pistachio_ringbreaker/Reaxys/Reaxys_biocatalysis |
Top-N result to add to graph | 6 |
Feasible Synthetic Routes
Disclaimer and Information on In-Vitro Research Products
Please be aware that all articles and product information presented on BenchChem are intended solely for informational purposes. The products available for purchase on BenchChem are specifically designed for in-vitro studies, which are conducted outside of living organisms. In-vitro studies, derived from the Latin term "in glass," involve experiments performed in controlled laboratory settings using cells or tissues. It is important to note that these products are not categorized as medicines or drugs, and they have not received approval from the FDA for the prevention, treatment, or cure of any medical condition, ailment, or disease. We must emphasize that any form of bodily introduction of these products into humans or animals is strictly prohibited by law. It is essential to adhere to these guidelines to ensure compliance with legal and ethical standards in research and experimentation.