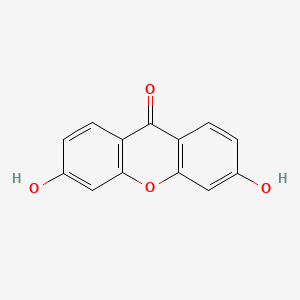
3,6-dihydroxy-9H-xanthen-9-one
Overview
Description
3,6-Dihydroxyxanthone is a derivative of xanthone, a class of organic compounds known for their diverse biological activities. Xanthones are characterized by a dibenzo-γ-pyrone structure, and 3,6-Dihydroxyxanthone specifically has hydroxyl groups at the 3rd and 6th positions. This compound has garnered attention due to its potential therapeutic properties, including anticancer and antioxidant activities .
Mechanism of Action
Target of Action
3,6-Dihydroxy-9H-xanthen-9-one is a xanthone derivative that has been shown to inhibit the growth of cancer cells in vitro .
Mode of Action
The mode of action of this compound involves inhibiting protein synthesis and DNA synthesis within the cell . This inhibition disrupts the normal functioning of the cell, leading to a decrease in cell proliferation and potentially leading to cell death .
Biochemical Pathways
The biosynthesis of this compound in plants involves the shikimate and the acetate pathways . These pathways originate in plastids and endoplasmic reticulum, respectively . The pathway continues following three alternative routes, two phenylalanine-dependent and one phenylalanine-independent . All three routes lead to the biosynthesis of 2,3′,4,6-tetrahydroxybenzophenone, which is the central intermediate .
Result of Action
The result of the action of this compound is a decrease in cell proliferation, potentially leading to cell death . This is due to the compound’s ability to inhibit protein synthesis and DNA synthesis within the cell .
Action Environment
The action of this compound can be influenced by various environmental factors. For example, the compound’s lipophilicity can affect its interaction with biological membranes Additionally, factors such as pH, temperature, and the presence of other compounds can influence the compound’s stability, efficacy, and action
Biochemical Analysis
Biochemical Properties
3,6-Dihydroxy-9H-xanthen-9-one plays a significant role in biochemical reactions due to its ability to interact with multiple enzymes and proteins. It has been shown to inhibit the activity of α-glucosidase, an enzyme involved in carbohydrate metabolism . This inhibition can lead to a decrease in blood glucose levels, making it a potential candidate for managing diabetes. Additionally, this compound interacts with various proteins involved in cell signaling pathways, such as kinases and phosphatases, modulating their activity and influencing cellular responses .
Cellular Effects
The effects of this compound on cellular processes are profound. It has been observed to induce apoptosis in cancer cells by activating caspases, a family of proteases involved in programmed cell death . This compound also affects cell signaling pathways, such as the MAPK and PI3K/Akt pathways, which are crucial for cell proliferation and survival . Furthermore, this compound influences gene expression by modulating transcription factors, leading to changes in the expression of genes involved in cell cycle regulation and apoptosis .
Molecular Mechanism
At the molecular level, this compound exerts its effects through various mechanisms. It binds to the active sites of enzymes, inhibiting their activity and altering metabolic pathways . For instance, its interaction with α-glucosidase involves hydrogen bonding and hydrophobic interactions, leading to enzyme inhibition . Additionally, this compound can activate or inhibit transcription factors, resulting in changes in gene expression . These molecular interactions highlight the compound’s potential as a therapeutic agent.
Temporal Effects in Laboratory Settings
In laboratory settings, the effects of this compound have been studied over various time periods. The compound is relatively stable under standard laboratory conditions, but its activity can decrease over time due to degradation . Long-term studies have shown that prolonged exposure to this compound can lead to sustained inhibition of target enzymes and persistent changes in gene expression . These temporal effects are crucial for understanding the compound’s potential therapeutic applications.
Dosage Effects in Animal Models
The effects of this compound vary with different dosages in animal models. At low doses, the compound has been shown to exert beneficial effects, such as reducing blood glucose levels and inhibiting tumor growth . At higher doses, toxic effects can occur, including liver and kidney damage . These findings highlight the importance of determining the optimal dosage for therapeutic use to minimize adverse effects while maximizing benefits.
Metabolic Pathways
This compound is involved in several metabolic pathways. It is metabolized by liver enzymes, including cytochrome P450, which convert it into various metabolites . These metabolites can have different biological activities, contributing to the overall effects of the compound . Additionally, this compound can influence metabolic flux by inhibiting key enzymes in carbohydrate and lipid metabolism .
Transport and Distribution
Within cells and tissues, this compound is transported and distributed through various mechanisms. It can diffuse across cell membranes due to its lipophilic nature and is also transported by specific transporters . Once inside the cell, the compound can accumulate in certain organelles, such as the mitochondria and nucleus, where it exerts its effects . The distribution of this compound within tissues is influenced by factors such as blood flow and tissue permeability .
Subcellular Localization
The subcellular localization of this compound is critical for its activity. The compound has been found to localize in the mitochondria, where it can induce apoptosis by disrupting the mitochondrial membrane potential . It also accumulates in the nucleus, where it can interact with DNA and transcription factors to modulate gene expression . These localization patterns are essential for understanding the compound’s mechanism of action and potential therapeutic applications.
Preparation Methods
Synthetic Routes and Reaction Conditions: 3,6-Dihydroxyxanthone can be synthesized from 2,6-dihydroxybenzoic acid. The process involves the reaction of 2,6-dihydroxybenzoic acid with phloroglucinol, resorcinol, or pyrogallol under specific conditions . The reaction typically requires a dehydrating agent such as acetic anhydride and a catalyst like zinc chloride or phosphoryl chloride to facilitate the formation of the xanthone structure .
Industrial Production Methods: Industrial production of 3,6-Dihydroxyxanthone follows similar synthetic routes but on a larger scale. The process involves optimizing reaction conditions to maximize yield and purity. Techniques such as microwave heating have been employed to enhance reaction efficiency and reduce production time .
Chemical Reactions Analysis
Types of Reactions: 3,6-Dihydroxyxanthone undergoes various chemical reactions, including:
Oxidation: The hydroxyl groups can be oxidized to form quinones.
Reduction: The compound can be reduced to form dihydro derivatives.
Substitution: The hydroxyl groups can participate in substitution reactions to form ethers or esters.
Common Reagents and Conditions:
Oxidation: Common oxidizing agents include potassium permanganate and chromium trioxide.
Reduction: Reducing agents such as sodium borohydride or lithium aluminum hydride are used.
Substitution: Reagents like alkyl halides or acyl chlorides are employed in the presence of a base.
Major Products:
Oxidation: Quinone derivatives.
Reduction: Dihydro derivatives.
Substitution: Ethers and esters.
Scientific Research Applications
Chemistry: Used as a building block for synthesizing more complex molecules.
Medicine: Explored for its anticancer activity, particularly against cell lines such as WiDr, MCF-7, and HeLa.
Comparison with Similar Compounds
- 1,3,8-Trihydroxyxanthone
- 1,6-Dihydroxyxanthone
- 1,5,6-Trihydroxyxanthone
Comparison:
- 1,3,8-Trihydroxyxanthone: Similar antioxidant properties but different hydroxyl group positions.
- 1,6-Dihydroxyxanthone: Shares the same number of hydroxyl groups but at different positions, leading to variations in biological activity.
- 1,5,6-Trihydroxyxanthone: Contains an additional hydroxyl group, which may enhance its antioxidant and anticancer activities .
3,6-Dihydroxyxanthone stands out due to its specific hydroxyl group positions, which contribute to its unique biological activities and potential therapeutic applications.
Properties
IUPAC Name |
3,6-dihydroxyxanthen-9-one | |
---|---|---|
Source | PubChem | |
URL | https://pubchem.ncbi.nlm.nih.gov | |
Description | Data deposited in or computed by PubChem | |
InChI |
InChI=1S/C13H8O4/c14-7-1-3-9-11(5-7)17-12-6-8(15)2-4-10(12)13(9)16/h1-6,14-15H | |
Source | PubChem | |
URL | https://pubchem.ncbi.nlm.nih.gov | |
Description | Data deposited in or computed by PubChem | |
InChI Key |
POARTHFLPKAZBQ-UHFFFAOYSA-N | |
Source | PubChem | |
URL | https://pubchem.ncbi.nlm.nih.gov | |
Description | Data deposited in or computed by PubChem | |
Canonical SMILES |
C1=CC2=C(C=C1O)OC3=C(C2=O)C=CC(=C3)O | |
Source | PubChem | |
URL | https://pubchem.ncbi.nlm.nih.gov | |
Description | Data deposited in or computed by PubChem | |
Molecular Formula |
C13H8O4 | |
Source | PubChem | |
URL | https://pubchem.ncbi.nlm.nih.gov | |
Description | Data deposited in or computed by PubChem | |
Molecular Weight |
228.20 g/mol | |
Source | PubChem | |
URL | https://pubchem.ncbi.nlm.nih.gov | |
Description | Data deposited in or computed by PubChem | |
Synthesis routes and methods
Procedure details
Retrosynthesis Analysis
AI-Powered Synthesis Planning: Our tool employs the Template_relevance Pistachio, Template_relevance Bkms_metabolic, Template_relevance Pistachio_ringbreaker, Template_relevance Reaxys, Template_relevance Reaxys_biocatalysis model, leveraging a vast database of chemical reactions to predict feasible synthetic routes.
One-Step Synthesis Focus: Specifically designed for one-step synthesis, it provides concise and direct routes for your target compounds, streamlining the synthesis process.
Accurate Predictions: Utilizing the extensive PISTACHIO, BKMS_METABOLIC, PISTACHIO_RINGBREAKER, REAXYS, REAXYS_BIOCATALYSIS database, our tool offers high-accuracy predictions, reflecting the latest in chemical research and data.
Strategy Settings
Precursor scoring | Relevance Heuristic |
---|---|
Min. plausibility | 0.01 |
Model | Template_relevance |
Template Set | Pistachio/Bkms_metabolic/Pistachio_ringbreaker/Reaxys/Reaxys_biocatalysis |
Top-N result to add to graph | 6 |
Feasible Synthetic Routes
Q1: How does 3,6-dihydroxy-9H-xanthen-9-one interact with its target to exert its antimalarial activity?
A1: While the exact mechanism of action of this compound is still under investigation, molecular docking studies suggest it targets the Plasmodium falciparum dihydrofolate reductase-thymidylate synthase (PfDHFR-TS) enzyme. [] This enzyme is crucial for parasite DNA synthesis. The docking studies indicate that this compound interacts with key amino acids in the PfDHFR-TS active site, including Ala16, Ser108, Phe58, Asp54, and Leu46. [] These interactions likely inhibit enzyme activity, ultimately disrupting parasite growth and survival.
Q2: What is the structure and in vitro activity of this compound?
A2: this compound is a xanthone derivative with the molecular formula C13H8O4. [] While the paper doesn't explicitly state the molecular weight or spectroscopic data, it does highlight its potent in vitro antimalarial activity. It exhibited an IC50 value of 0.71 µM against the chloroquine-sensitive 3D7 strain of Plasmodium falciparum. [] This indicates a strong inhibitory effect on parasite growth in laboratory settings.
Q3: How does the structure of this compound relate to its antimalarial activity?
A3: The research utilized Quantitative Structure-Activity Relationship (QSAR) analysis to understand the relationship between the structure of xanthone derivatives and their antimalarial activity. [] The QSAR model developed identified specific molecular descriptors, including charges on specific atoms within the xanthone structure, as significant contributors to antimalarial potency. [] This suggests that modifications altering these electronic properties could impact the compound's interaction with PfDHFR-TS and its subsequent efficacy. Further research exploring structural analogs and their activities could solidify these structure-activity relationships.
Disclaimer and Information on In-Vitro Research Products
Please be aware that all articles and product information presented on BenchChem are intended solely for informational purposes. The products available for purchase on BenchChem are specifically designed for in-vitro studies, which are conducted outside of living organisms. In-vitro studies, derived from the Latin term "in glass," involve experiments performed in controlled laboratory settings using cells or tissues. It is important to note that these products are not categorized as medicines or drugs, and they have not received approval from the FDA for the prevention, treatment, or cure of any medical condition, ailment, or disease. We must emphasize that any form of bodily introduction of these products into humans or animals is strictly prohibited by law. It is essential to adhere to these guidelines to ensure compliance with legal and ethical standards in research and experimentation.