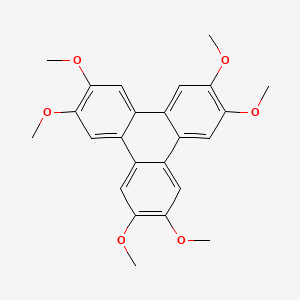
2,3,6,7,10,11-Hexamethoxytriphenylene
Overview
Description
2,3,6,7,10,11-Hexamethoxytriphenylene is an organic compound with the molecular formula C24H24O6 and a molecular weight of 408.44 g/mol . It is a fully conjugated triphenylene ligand, characterized by its planar disc-shaped structure and six methoxy groups at the ortho-positions . This compound is known for its high reversibility in electrochemical redox reactions, making it a valuable material in various scientific and industrial applications .
Biochemical Analysis
Biochemical Properties
2,3,6,7,10,11-Hexamethoxytriphenylene plays a significant role in biochemical reactions, particularly in the context of its interactions with enzymes and proteins. It has been observed to interact with various biomolecules, influencing their activity and stability. For instance, the compound can act as a substrate or inhibitor for certain enzymes, thereby modulating their catalytic functions. The nature of these interactions is often dictated by the structural compatibility between this compound and the active sites of the enzymes or proteins it interacts with .
Cellular Effects
The effects of this compound on cellular processes are diverse and multifaceted. It has been shown to influence cell signaling pathways, gene expression, and cellular metabolism. For example, the compound can modulate the activity of key signaling molecules, leading to alterations in downstream cellular responses. Additionally, this compound can affect the expression of specific genes, thereby impacting cellular functions such as proliferation, differentiation, and apoptosis .
Molecular Mechanism
At the molecular level, this compound exerts its effects through various mechanisms. One of the primary modes of action involves binding interactions with biomolecules, such as enzymes and receptors. These interactions can lead to enzyme inhibition or activation, depending on the nature of the binding. Furthermore, this compound can influence gene expression by interacting with transcription factors or other regulatory proteins, thereby modulating the transcriptional activity of target genes .
Temporal Effects in Laboratory Settings
In laboratory settings, the effects of this compound can change over time. The compound’s stability and degradation are critical factors that influence its long-term effects on cellular function. Studies have shown that this compound can undergo degradation under certain conditions, leading to the formation of metabolites with distinct biological activities. These temporal changes can impact the compound’s efficacy and safety in both in vitro and in vivo studies .
Dosage Effects in Animal Models
The effects of this compound vary with different dosages in animal models. At lower doses, the compound may exhibit beneficial effects, such as enhancing cellular functions or protecting against oxidative stress. At higher doses, this compound can induce toxic or adverse effects, including cellular damage and apoptosis. These dosage-dependent effects highlight the importance of determining the optimal therapeutic window for the compound’s use in biomedical applications .
Metabolic Pathways
This compound is involved in various metabolic pathways, interacting with enzymes and cofactors that facilitate its biotransformation. The compound can undergo phase I and phase II metabolic reactions, leading to the formation of metabolites that may possess distinct biological activities. These metabolic pathways can influence the compound’s pharmacokinetics and pharmacodynamics, affecting its overall efficacy and safety profile .
Transport and Distribution
The transport and distribution of this compound within cells and tissues are critical determinants of its biological activity. The compound can interact with specific transporters or binding proteins that facilitate its uptake and distribution. Additionally, this compound can accumulate in certain cellular compartments, influencing its localization and activity. Understanding these transport and distribution mechanisms is essential for optimizing the compound’s therapeutic potential .
Subcellular Localization
The subcellular localization of this compound plays a crucial role in its activity and function. The compound can be directed to specific cellular compartments or organelles through targeting signals or post-translational modifications. This localization can impact the compound’s interactions with biomolecules and its overall biological effects. For instance, this compound may localize to the nucleus, where it can influence gene expression, or to the mitochondria, where it can affect cellular metabolism .
Preparation Methods
Synthetic Routes and Reaction Conditions
2,3,6,7,10,11-Hexamethoxytriphenylene can be synthesized through the oxidative trimerization of 1,2-dimethoxybenzene using transition metal compounds such as iron(III) chloride . The reaction typically involves the following steps:
Oxidative Trimerization: 1,2-dimethoxybenzene is oxidized using iron(III) chloride to form this compound.
Demethylation: The hexamethoxy compound can be further demethylated using strong Lewis acids like boron tribromide (BBr3) to yield hexahydroxytriphenylene.
Industrial Production Methods
The industrial production of this compound follows similar synthetic routes but on a larger scale. The process involves careful control of reaction conditions to ensure high yields and purity of the final product .
Chemical Reactions Analysis
Types of Reactions
2,3,6,7,10,11-Hexamethoxytriphenylene undergoes various chemical reactions, including:
Oxidation: The compound can participate in electrochemical redox reactions, which are highly reversible.
Demethylation: Strong Lewis acids can demethylate the compound to form hexahydroxytriphenylene.
Common Reagents and Conditions
Oxidation: Iron(III) chloride is commonly used for the oxidative trimerization of 1,2-dimethoxybenzene.
Demethylation: Boron tribromide (BBr3) is used for the demethylation process.
Major Products Formed
Hexahydroxytriphenylene: Formed through the demethylation of this compound.
Scientific Research Applications
2,3,6,7,10,11-Hexamethoxytriphenylene has a wide range of applications in scientific research, including:
Mechanism of Action
The mechanism of action of 2,3,6,7,10,11-hexamethoxytriphenylene involves its ability to undergo reversible redox reactions. The compound’s planar structure and conjugated system facilitate efficient electron transfer, making it an effective material for applications in batteries and other electrochemical devices . The molecular targets and pathways involved include interactions with various metal ions and organic molecules, leading to the formation of stable complexes .
Comparison with Similar Compounds
2,3,6,7,10,11-Hexamethoxytriphenylene can be compared with other similar compounds, such as:
Triphenylene: The parent compound of this compound, lacking the methoxy groups.
Hexahydroxytriphenylene: The demethylated form of this compound, with hydroxyl groups instead of methoxy groups.
1,3,5-Tris(4-carboxyphenyl)benzene: Another triphenylene derivative used in the synthesis of COFs.
The uniqueness of this compound lies in its high reversibility in redox reactions and its ability to form stable complexes, making it a valuable material in various scientific and industrial applications .
Properties
IUPAC Name |
2,3,6,7,10,11-hexamethoxytriphenylene | |
---|---|---|
Source | PubChem | |
URL | https://pubchem.ncbi.nlm.nih.gov | |
Description | Data deposited in or computed by PubChem | |
InChI |
InChI=1S/C24H24O6/c1-25-19-7-13-14(8-20(19)26-2)16-10-22(28-4)24(30-6)12-18(16)17-11-23(29-5)21(27-3)9-15(13)17/h7-12H,1-6H3 | |
Source | PubChem | |
URL | https://pubchem.ncbi.nlm.nih.gov | |
Description | Data deposited in or computed by PubChem | |
InChI Key |
TXROZCSFVVIBFI-UHFFFAOYSA-N | |
Source | PubChem | |
URL | https://pubchem.ncbi.nlm.nih.gov | |
Description | Data deposited in or computed by PubChem | |
Canonical SMILES |
COC1=C(C=C2C(=C1)C3=CC(=C(C=C3C4=CC(=C(C=C24)OC)OC)OC)OC)OC | |
Source | PubChem | |
URL | https://pubchem.ncbi.nlm.nih.gov | |
Description | Data deposited in or computed by PubChem | |
Molecular Formula |
C24H24O6 | |
Source | PubChem | |
URL | https://pubchem.ncbi.nlm.nih.gov | |
Description | Data deposited in or computed by PubChem | |
DSSTOX Substance ID |
DTXSID70404819 | |
Record name | 2,3,6,7,10,11-Hexamethoxytriphenylene | |
Source | EPA DSSTox | |
URL | https://comptox.epa.gov/dashboard/DTXSID70404819 | |
Description | DSSTox provides a high quality public chemistry resource for supporting improved predictive toxicology. | |
Molecular Weight |
408.4 g/mol | |
Source | PubChem | |
URL | https://pubchem.ncbi.nlm.nih.gov | |
Description | Data deposited in or computed by PubChem | |
CAS No. |
808-57-1 | |
Record name | 2,3,6,7,10,11-Hexamethoxytriphenylene | |
Source | EPA DSSTox | |
URL | https://comptox.epa.gov/dashboard/DTXSID70404819 | |
Description | DSSTox provides a high quality public chemistry resource for supporting improved predictive toxicology. | |
Synthesis routes and methods I
Procedure details
Synthesis routes and methods II
Procedure details
Synthesis routes and methods III
Procedure details
Synthesis routes and methods IV
Procedure details
Retrosynthesis Analysis
AI-Powered Synthesis Planning: Our tool employs the Template_relevance Pistachio, Template_relevance Bkms_metabolic, Template_relevance Pistachio_ringbreaker, Template_relevance Reaxys, Template_relevance Reaxys_biocatalysis model, leveraging a vast database of chemical reactions to predict feasible synthetic routes.
One-Step Synthesis Focus: Specifically designed for one-step synthesis, it provides concise and direct routes for your target compounds, streamlining the synthesis process.
Accurate Predictions: Utilizing the extensive PISTACHIO, BKMS_METABOLIC, PISTACHIO_RINGBREAKER, REAXYS, REAXYS_BIOCATALYSIS database, our tool offers high-accuracy predictions, reflecting the latest in chemical research and data.
Strategy Settings
Precursor scoring | Relevance Heuristic |
---|---|
Min. plausibility | 0.01 |
Model | Template_relevance |
Template Set | Pistachio/Bkms_metabolic/Pistachio_ringbreaker/Reaxys/Reaxys_biocatalysis |
Top-N result to add to graph | 6 |
Feasible Synthetic Routes
Q1: What is the molecular structure and formula of 2,3,6,7,10,11-Hexamethoxytriphenylene?
A1: this compound (HMTP) comprises a central triphenylene core, a polyaromatic hydrocarbon, decorated with six methoxy (-OCH3) groups. Its molecular formula is C24H24O6. [, , , ]
Q2: How does HMTP interact with surfaces like silver (Ag(111)) and graphene?
A2: HMTP exhibits weak interactions with graphene, forming a commensurate close-packed hexagonal network on graphene/Ir(111). [] Conversely, on Ag(111) surfaces, HMTP interacts weakly while its acceptor counterpart, HATCN, demonstrates strong interactions, leading to charge transfer and surface buckling. Interestingly, mixing HMTP and HATCN results in strong hybridization and the emergence of a common unoccupied molecular orbital, signifying potential for charge-transfer complex formation. []
Q3: Can HMTP be used as a component in organic electronic devices?
A3: Research suggests that HMTP, as an electron donor, can form charge-transfer complexes with acceptor molecules like HATCN. [] These complexes are crucial for developing organic electronic devices like organic solar cells and transistors.
Q4: Are there alternative methods to synthesize HMTP besides traditional chemical routes?
A4: Yes, electrochemical methods offer a greener approach to synthesizing HMTP derivatives. Anodic oxidation of catechol ketals, followed by acidic hydrolysis, can yield high-quality HMTP. This method minimizes metal waste and avoids harsh reaction conditions. []
Q5: What is known about the crystal structure of HMTP?
A5: HMTP crystallizes differently than expected for typical discotic columnar mesogens. Instead of stacking based on the aromatic core, its crystal structure is primarily governed by the arrangement of alkyl groups. [] This insight highlights the importance of peripheral groups in influencing the self-assembly and ultimately the material properties of HMTP.
Q6: What are the challenges associated with studying HMTP adsorption on alkali halide surfaces?
A7: Studying molecular adsorption on bulk insulator surfaces like KBr(001) is challenging due to the limited number of molecules suitable for such investigations. HMTP, with its flat aromatic core and peripheral polar groups, was specifically designed to enhance interactions with KBr(001) and minimize molecular diffusion at room temperature. []
Q7: How does the structure of HMTP contribute to its properties and potential applications?
A8: The planar aromatic core of HMTP facilitates π-π stacking interactions, which are crucial for charge transport in organic electronics. [] Additionally, the six methoxy groups enhance solubility and influence the molecule's self-assembly behavior, impacting its potential applications in various fields. []
Disclaimer and Information on In-Vitro Research Products
Please be aware that all articles and product information presented on BenchChem are intended solely for informational purposes. The products available for purchase on BenchChem are specifically designed for in-vitro studies, which are conducted outside of living organisms. In-vitro studies, derived from the Latin term "in glass," involve experiments performed in controlled laboratory settings using cells or tissues. It is important to note that these products are not categorized as medicines or drugs, and they have not received approval from the FDA for the prevention, treatment, or cure of any medical condition, ailment, or disease. We must emphasize that any form of bodily introduction of these products into humans or animals is strictly prohibited by law. It is essential to adhere to these guidelines to ensure compliance with legal and ethical standards in research and experimentation.