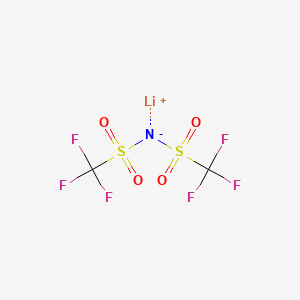
Lithium bis((trifluoromethyl)sulfonyl)azanide
Overview
Description
Bis(trifluoromethane)sulfonimide lithium salt is a synthetic reagent.
Biochemical Analysis
Biochemical Properties
Lithium bis((trifluoromethyl)sulfonyl)azanide plays a significant role in biochemical reactions, particularly in the context of its interactions with enzymes, proteins, and other biomolecules. The compound’s anion, bistriflimide, coordinates weakly with cations, which can influence the activity of various enzymes and proteins. For instance, it has been shown to interact with carbonyl groups in certain covalent organic frameworks, forming hydrogen bonds that can affect the transfer number of lithium ions . These interactions can modulate the activity of enzymes involved in metabolic pathways, thereby influencing biochemical reactions.
Cellular Effects
This compound has notable effects on various types of cells and cellular processes. It influences cell function by affecting cell signaling pathways, gene expression, and cellular metabolism. The compound’s high solubility and ability to coordinate weakly with cations allow it to penetrate cell membranes and interact with intracellular components. This can lead to alterations in cell signaling pathways, such as those involving calcium ions, and changes in gene expression that impact cellular metabolism .
Molecular Mechanism
The molecular mechanism of action of this compound involves its interactions with biomolecules at the molecular level. The compound’s anion, bistriflimide, coordinates weakly with cations, which can lead to enzyme inhibition or activation. For example, it can inhibit enzymes involved in the degradation of certain metabolites, thereby altering metabolic flux. Additionally, this compound can bind to specific proteins, leading to changes in their conformation and activity .
Temporal Effects in Laboratory Settings
In laboratory settings, the effects of this compound can change over time. The compound’s stability and degradation are critical factors that influence its long-term effects on cellular function. Studies have shown that this compound can cause damage to the nervous system through prolonged or repeated exposure . Additionally, its high solubility in water ensures that it remains active in aqueous environments, maintaining its biochemical effects over extended periods.
Dosage Effects in Animal Models
The effects of this compound vary with different dosages in animal models. At low doses, the compound can enhance the activity of certain enzymes and proteins, leading to beneficial effects on cellular function. At high doses, this compound can cause toxic or adverse effects, such as severe skin burns and eye damage . Threshold effects have been observed, indicating that there is a specific dosage range within which the compound exerts its optimal effects without causing toxicity.
Metabolic Pathways
This compound is involved in various metabolic pathways, interacting with enzymes and cofactors that regulate metabolic flux. The compound’s anion, bistriflimide, can inhibit enzymes involved in the degradation of certain metabolites, leading to changes in metabolite levels. Additionally, this compound can affect the activity of enzymes that regulate the synthesis and breakdown of key biomolecules, thereby influencing overall metabolic pathways .
Transport and Distribution
Within cells and tissues, this compound is transported and distributed through interactions with transporters and binding proteins. The compound’s high solubility in water facilitates its movement across cell membranes and its accumulation in specific cellular compartments. This can lead to localized effects on cellular function, depending on the concentration and distribution of this compound within the cell .
Subcellular Localization
The subcellular localization of this compound is influenced by targeting signals and post-translational modifications that direct it to specific compartments or organelles. The compound can accumulate in organelles such as mitochondria and lysosomes, where it can exert its effects on cellular metabolism and function. This localization is critical for its activity, as it allows this compound to interact with specific biomolecules and modulate their activity .
Properties
CAS No. |
90076-65-6 |
---|---|
Molecular Formula |
C2HF6LiNO4S2 |
Molecular Weight |
288.1 g/mol |
IUPAC Name |
lithium;bis(trifluoromethylsulfonyl)azanide |
InChI |
InChI=1S/C2HF6NO4S2.Li/c3-1(4,5)14(10,11)9-15(12,13)2(6,7)8;/h9H; |
InChI Key |
UGEOMRBXRFUYJH-UHFFFAOYSA-N |
SMILES |
[Li+].C(F)(F)(F)S(=O)(=O)[N-]S(=O)(=O)C(F)(F)F |
Canonical SMILES |
[Li].C(F)(F)(F)S(=O)(=O)NS(=O)(=O)C(F)(F)F |
90076-65-6 | |
physical_description |
PelletsLargeCrystals |
Pictograms |
Corrosive; Acute Toxic; Health Hazard |
Origin of Product |
United States |
Synthesis routes and methods
Procedure details
Retrosynthesis Analysis
AI-Powered Synthesis Planning: Our tool employs the Template_relevance Pistachio, Template_relevance Bkms_metabolic, Template_relevance Pistachio_ringbreaker, Template_relevance Reaxys, Template_relevance Reaxys_biocatalysis model, leveraging a vast database of chemical reactions to predict feasible synthetic routes.
One-Step Synthesis Focus: Specifically designed for one-step synthesis, it provides concise and direct routes for your target compounds, streamlining the synthesis process.
Accurate Predictions: Utilizing the extensive PISTACHIO, BKMS_METABOLIC, PISTACHIO_RINGBREAKER, REAXYS, REAXYS_BIOCATALYSIS database, our tool offers high-accuracy predictions, reflecting the latest in chemical research and data.
Strategy Settings
Precursor scoring | Relevance Heuristic |
---|---|
Min. plausibility | 0.01 |
Model | Template_relevance |
Template Set | Pistachio/Bkms_metabolic/Pistachio_ringbreaker/Reaxys/Reaxys_biocatalysis |
Top-N result to add to graph | 6 |
Feasible Synthetic Routes
Q1: Why is LiTFSI considered a promising electrolyte salt for lithium-ion batteries?
A1: LiTFSI exhibits several advantageous properties over conventional lithium hexafluorophosphate (LiPF6), including:
- Higher thermal stability: This leads to improved safety and performance at elevated temperatures. [, ]
- Resistance to hydrolysis: This enhances moisture resistance, simplifying battery manufacturing and extending shelf life. []
- High ionic conductivity: LiTFSI-based electrolytes facilitate efficient ion transport, enabling high-rate performance. [, , , ]
Q2: How does LiTFSI interact with electrode materials like silicon and impact battery performance?
A3: In silicon anodes, the interaction between LiTFSI and the silicon surface can lead to the formation of an unstable solid electrolyte interphase (SEI), resulting in capacity fading. Researchers are exploring strategies like incorporating electrolyte additives and novel binders to mitigate these issues. [, ]
Q3: What strategies can mitigate aluminum corrosion caused by LiTFSI in high-voltage batteries?
A3: While LiTFSI offers benefits, it can corrode aluminum current collectors at high voltages. Strategies to circumvent this include:
- Low-voltage operation: Limiting the cell voltage below the threshold for aluminum corrosion. []
- Salt additives: Incorporating additives like lithium tetrafluoroborate (LiBF4) or lithium difluoro(oxalato)borate (LiDFOB) to suppress corrosion. []
Q4: What is the role of LiTFSI in gel polymer electrolytes (GPEs)?
A6: In GPEs, LiTFSI serves as the primary source of charge carriers. It dissolves in a polymer matrix and dissociates into Li+ and TFSI- ions, enabling ionic conductivity. The properties of the GPE, such as ionic conductivity and electrochemical stability, depend on the polymer-salt interactions and the concentration of LiTFSI. []
Q5: How does the presence of LiTFSI affect the polymerization of methyl methacrylate (MMA)?
A7: LiTFSI, typically considered a poor initiator for MMA polymerization, can be used in conjunction with fluoroethylene carbonate (FEC). FEC's high dielectric constant promotes LiTFSI dissociation, enhancing its ability to initiate MMA polymerization. This approach facilitates the creation of novel polymer electrolytes with desirable properties for lithium metal batteries. []
Q6: What is the molecular formula and weight of LiTFSI?
A6: The molecular formula of LiTFSI is C2F6LiNO4S2, and its molecular weight is 287.09 g/mol.
Q7: What spectroscopic techniques are commonly employed to characterize LiTFSI?
A7: Common techniques include:
- Fourier-transform infrared spectroscopy (FTIR): This method helps identify functional groups and analyze interactions between LiTFSI and other components in electrolytes. []
- Nuclear magnetic resonance (NMR) spectroscopy: NMR provides insights into the solvation structure and dynamics of LiTFSI in various solvents. []
- X-ray photoelectron spectroscopy (XPS): This surface-sensitive technique is valuable for analyzing the composition and chemical states of SEI layers formed in the presence of LiTFSI. [, ]
Q8: What is the role of additives like fluoroethylene carbonate (FEC) in LiTFSI-based electrolytes?
A11: FEC acts as a solid electrolyte interphase (SEI)-forming additive, contributing to a more stable and robust SEI layer on electrode surfaces. This, in turn, improves the cycling performance and lifespan of the battery. []
Q9: How does the thermal stability of LiTFSI contribute to battery safety?
A13: LiTFSI's higher thermal stability compared to LiPF6 reduces the risk of electrolyte decomposition and thermal runaway at elevated temperatures, contributing to safer battery operation. [, ]
Q10: Are there any environmental concerns associated with LiTFSI?
A14: While LiTFSI offers advantages over LiPF6, its potential environmental impact requires further investigation. Research on its biodegradability, ecotoxicological effects, and sustainable disposal methods is crucial. []
Q11: How is computational chemistry employed in LiTFSI research?
A11: Computational methods such as molecular dynamics (MD) simulations and density functional theory (DFT) calculations are essential for:
- Understanding ion transport mechanisms: Simulations provide insights into the movement of Li+ and TFSI- ions in different electrolyte systems. [, , ]
- Predicting electrolyte properties: Computational models help predict ionic conductivity, viscosity, and other critical parameters based on electrolyte composition. []
- Designing novel electrolyte materials: Simulations guide the development of new LiTFSI-based electrolytes with improved performance and safety characteristics. [, ]
Disclaimer and Information on In-Vitro Research Products
Please be aware that all articles and product information presented on BenchChem are intended solely for informational purposes. The products available for purchase on BenchChem are specifically designed for in-vitro studies, which are conducted outside of living organisms. In-vitro studies, derived from the Latin term "in glass," involve experiments performed in controlled laboratory settings using cells or tissues. It is important to note that these products are not categorized as medicines or drugs, and they have not received approval from the FDA for the prevention, treatment, or cure of any medical condition, ailment, or disease. We must emphasize that any form of bodily introduction of these products into humans or animals is strictly prohibited by law. It is essential to adhere to these guidelines to ensure compliance with legal and ethical standards in research and experimentation.