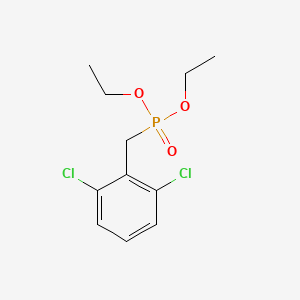
Diethyl (2,6-dichlorobenzyl)phosphonate
Overview
Description
Diethyl (2,6-dichlorobenzyl)phosphonate is a chemical compound with the molecular formula C11H15Cl2O3P and a molecular weight of 297.12. It is also known by its IUPAC name, diethyl 2,6-dichlorobenzylphosphonate . This compound is characterized by the presence of two chlorine atoms and a diethoxyphosphorylmethyl group attached to a benzene ring, making it a versatile molecule in various chemical reactions and applications.
Preparation Methods
Synthetic Routes and Reaction Conditions
The synthesis of Diethyl (2,6-dichlorobenzyl)phosphonate typically involves the reaction of 1,3-dichlorobenzene with diethyl phosphite in the presence of a base. The reaction conditions often include heating the mixture to a specific temperature to facilitate the formation of the desired product .
Industrial Production Methods
In industrial settings, the production of this compound may involve large-scale reactions using similar reagents and conditions as in laboratory synthesis. The process is optimized for higher yields and purity, often involving purification steps such as distillation or recrystallization to obtain the final product .
Chemical Reactions Analysis
Types of Reactions
Diethyl (2,6-dichlorobenzyl)phosphonate undergoes various chemical reactions, including:
Substitution Reactions: The chlorine atoms can be substituted with other functional groups using appropriate reagents.
Oxidation and Reduction Reactions: The compound can undergo oxidation or reduction under specific conditions to form different products.
Common Reagents and Conditions
Substitution Reactions: Common reagents include nucleophiles such as amines or thiols, often under basic conditions.
Oxidation Reactions: Oxidizing agents like potassium permanganate or hydrogen peroxide can be used.
Reduction Reactions: Reducing agents such as lithium aluminum hydride or sodium borohydride are commonly employed.
Major Products Formed
The major products formed from these reactions depend on the specific reagents and conditions used. For example, substitution reactions can yield various substituted benzene derivatives, while oxidation and reduction reactions can lead to the formation of different phosphonate compounds .
Scientific Research Applications
Diethyl (2,6-dichlorobenzyl)phosphonate is utilized in various scientific research applications due to its unique chemical properties:
Chemistry: It serves as a precursor in the synthesis of other complex organic molecules.
Biology: The compound is used in studies involving enzyme inhibition and protein interactions.
Medicine: Research explores its potential as a pharmacological agent in drug development.
Industry: It finds applications in the production of specialty chemicals and materials.
Mechanism of Action
The mechanism of action of Diethyl (2,6-dichlorobenzyl)phosphonate involves its interaction with specific molecular targets and pathways. The compound can act as an inhibitor or activator of certain enzymes, affecting biochemical processes within cells. The exact molecular targets and pathways depend on the specific application and context of its use .
Comparison with Similar Compounds
Similar Compounds
- Diethyl 2,4-dichlorobenzylphosphonate
- Diethyl 2,5-dichlorobenzylphosphonate
- Diethyl 2,3-dichlorobenzylphosphonate
Uniqueness
Diethyl (2,6-dichlorobenzyl)phosphonate is unique due to the specific positioning of the chlorine atoms and the diethoxyphosphorylmethyl group on the benzene ring. This unique structure imparts distinct chemical reactivity and properties, making it valuable in various research and industrial applications .
Properties
IUPAC Name |
1,3-dichloro-2-(diethoxyphosphorylmethyl)benzene | |
---|---|---|
Source | PubChem | |
URL | https://pubchem.ncbi.nlm.nih.gov | |
Description | Data deposited in or computed by PubChem | |
InChI |
InChI=1S/C11H15Cl2O3P/c1-3-15-17(14,16-4-2)8-9-10(12)6-5-7-11(9)13/h5-7H,3-4,8H2,1-2H3 | |
Source | PubChem | |
URL | https://pubchem.ncbi.nlm.nih.gov | |
Description | Data deposited in or computed by PubChem | |
InChI Key |
WMVJGOZIAJRFLQ-UHFFFAOYSA-N | |
Source | PubChem | |
URL | https://pubchem.ncbi.nlm.nih.gov | |
Description | Data deposited in or computed by PubChem | |
Canonical SMILES |
CCOP(=O)(CC1=C(C=CC=C1Cl)Cl)OCC | |
Source | PubChem | |
URL | https://pubchem.ncbi.nlm.nih.gov | |
Description | Data deposited in or computed by PubChem | |
Molecular Formula |
C11H15Cl2O3P | |
Source | PubChem | |
URL | https://pubchem.ncbi.nlm.nih.gov | |
Description | Data deposited in or computed by PubChem | |
DSSTOX Substance ID |
DTXSID80383626 | |
Record name | Diethyl [(2,6-dichlorophenyl)methyl]phosphonate | |
Source | EPA DSSTox | |
URL | https://comptox.epa.gov/dashboard/DTXSID80383626 | |
Description | DSSTox provides a high quality public chemistry resource for supporting improved predictive toxicology. | |
Molecular Weight |
297.11 g/mol | |
Source | PubChem | |
URL | https://pubchem.ncbi.nlm.nih.gov | |
Description | Data deposited in or computed by PubChem | |
CAS No. |
63909-56-8 | |
Record name | Phosphonic acid, [(2,6-dichlorophenyl)methyl]-, diethyl ester | |
Source | CAS Common Chemistry | |
URL | https://commonchemistry.cas.org/detail?cas_rn=63909-56-8 | |
Description | CAS Common Chemistry is an open community resource for accessing chemical information. Nearly 500,000 chemical substances from CAS REGISTRY cover areas of community interest, including common and frequently regulated chemicals, and those relevant to high school and undergraduate chemistry classes. This chemical information, curated by our expert scientists, is provided in alignment with our mission as a division of the American Chemical Society. | |
Explanation | The data from CAS Common Chemistry is provided under a CC-BY-NC 4.0 license, unless otherwise stated. | |
Record name | Diethyl [(2,6-dichlorophenyl)methyl]phosphonate | |
Source | EPA DSSTox | |
URL | https://comptox.epa.gov/dashboard/DTXSID80383626 | |
Description | DSSTox provides a high quality public chemistry resource for supporting improved predictive toxicology. | |
Synthesis routes and methods I
Procedure details
Synthesis routes and methods II
Procedure details
Synthesis routes and methods III
Procedure details
Disclaimer and Information on In-Vitro Research Products
Please be aware that all articles and product information presented on BenchChem are intended solely for informational purposes. The products available for purchase on BenchChem are specifically designed for in-vitro studies, which are conducted outside of living organisms. In-vitro studies, derived from the Latin term "in glass," involve experiments performed in controlled laboratory settings using cells or tissues. It is important to note that these products are not categorized as medicines or drugs, and they have not received approval from the FDA for the prevention, treatment, or cure of any medical condition, ailment, or disease. We must emphasize that any form of bodily introduction of these products into humans or animals is strictly prohibited by law. It is essential to adhere to these guidelines to ensure compliance with legal and ethical standards in research and experimentation.