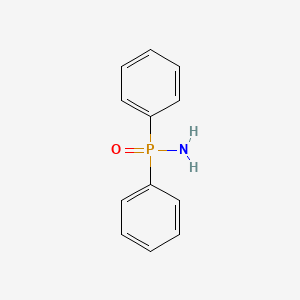
Diphenylphosphinamide
Overview
Description
Diphenylphosphinamide, also known as diphenyl phosphinic acid amide, is an organophosphorus compound with the molecular formula C12H12NOP and a molecular weight of 217.20 g/mol . It is a white to cream-colored solid that is soluble in chloroform, nitromethane, and nitrobenzene . This compound is used in various chemical reactions and has applications in scientific research.
Scientific Research Applications
Diphenylphosphinamide has several applications in scientific research:
Safety and Hazards
Mechanism of Action
Mode of Action
The mechanism of action of Diphenylphosphinamide has been investigated both theoretically and experimentally . According to these studies, a pre-reactive complex is formed between the alkyllithium and the phosphinamide . This complex evolves preferentially through NC (alpha)-metalation of the phosphinamide . The intramolecular nucleophilic addition of the carbanion to the ortho position of the aromatic ring leads to the dearomatized products .
Biochemical Pathways
Phosphonates, a class of compounds to which this compound belongs, are known to mimic the phosphates and carboxylates of biological molecules to potentially inhibit metabolic enzymes .
Preparation Methods
Synthetic Routes and Reaction Conditions
Diphenylphosphinamide can be synthesized through several methods. One common synthetic route involves the reaction of diphenylphosphinic chloride with ammonia or an amine under controlled conditions . The reaction typically proceeds as follows:
(C6H5)2P(O)Cl+NH3→(C6H5)2P(O)NH2+HCl
The reaction is carried out in an inert atmosphere, such as nitrogen or argon, to prevent the oxidation of the reactants and products .
Industrial Production Methods
Industrial production of this compound follows similar synthetic routes but on a larger scale. The reaction conditions are optimized to ensure high yield and purity of the product. The use of continuous flow reactors and automated systems helps in maintaining consistent reaction conditions and improving the efficiency of the production process .
Chemical Reactions Analysis
Types of Reactions
Diphenylphosphinamide undergoes various chemical reactions, including:
Oxidation: It can be oxidized to form diphenylphosphinic acid.
Reduction: It can be reduced to form diphenylphosphine.
Substitution: It can undergo nucleophilic substitution reactions with various nucleophiles.
Common Reagents and Conditions
Oxidation: Common oxidizing agents include hydrogen peroxide and potassium permanganate.
Reduction: Reducing agents such as lithium aluminum hydride or sodium borohydride are used.
Substitution: Nucleophiles like amines, alcohols, and thiols can react with this compound under mild conditions.
Major Products
Oxidation: Diphenylphosphinic acid.
Reduction: Diphenylphosphine.
Substitution: Various substituted phosphinamides depending on the nucleophile used.
Comparison with Similar Compounds
Similar Compounds
Diphenylphosphine: A related compound that can be synthesized by the reduction of diphenylphosphinamide.
Diphenylphosphinic acid: An oxidation product of this compound.
Phosphinic amides: A class of compounds with similar structures and reactivity.
Uniqueness
This compound is unique due to its ability to undergo a wide range of chemical reactions, making it a versatile reagent in organic synthesis. Its applications in the synthesis of enantioselective compounds and its role in biological studies further highlight its importance in scientific research .
Properties
IUPAC Name |
[amino(phenyl)phosphoryl]benzene | |
---|---|---|
Source | PubChem | |
URL | https://pubchem.ncbi.nlm.nih.gov | |
Description | Data deposited in or computed by PubChem | |
InChI |
InChI=1S/C12H12NOP/c13-15(14,11-7-3-1-4-8-11)12-9-5-2-6-10-12/h1-10H,(H2,13,14) | |
Source | PubChem | |
URL | https://pubchem.ncbi.nlm.nih.gov | |
Description | Data deposited in or computed by PubChem | |
InChI Key |
RIGIWEGXTTUCIQ-UHFFFAOYSA-N | |
Source | PubChem | |
URL | https://pubchem.ncbi.nlm.nih.gov | |
Description | Data deposited in or computed by PubChem | |
Canonical SMILES |
C1=CC=C(C=C1)P(=O)(C2=CC=CC=C2)N | |
Source | PubChem | |
URL | https://pubchem.ncbi.nlm.nih.gov | |
Description | Data deposited in or computed by PubChem | |
Molecular Formula |
C12H12NOP | |
Source | PubChem | |
URL | https://pubchem.ncbi.nlm.nih.gov | |
Description | Data deposited in or computed by PubChem | |
DSSTOX Substance ID |
DTXSID40357449 | |
Record name | Diphenylphosphinamide | |
Source | EPA DSSTox | |
URL | https://comptox.epa.gov/dashboard/DTXSID40357449 | |
Description | DSSTox provides a high quality public chemistry resource for supporting improved predictive toxicology. | |
Molecular Weight |
217.20 g/mol | |
Source | PubChem | |
URL | https://pubchem.ncbi.nlm.nih.gov | |
Description | Data deposited in or computed by PubChem | |
CAS No. |
5994-87-6 | |
Record name | Diphenylphosphinamide | |
Source | EPA DSSTox | |
URL | https://comptox.epa.gov/dashboard/DTXSID40357449 | |
Description | DSSTox provides a high quality public chemistry resource for supporting improved predictive toxicology. | |
Record name | Diphenylphosphinamide | |
Source | European Chemicals Agency (ECHA) | |
URL | https://echa.europa.eu/information-on-chemicals | |
Description | The European Chemicals Agency (ECHA) is an agency of the European Union which is the driving force among regulatory authorities in implementing the EU's groundbreaking chemicals legislation for the benefit of human health and the environment as well as for innovation and competitiveness. | |
Explanation | Use of the information, documents and data from the ECHA website is subject to the terms and conditions of this Legal Notice, and subject to other binding limitations provided for under applicable law, the information, documents and data made available on the ECHA website may be reproduced, distributed and/or used, totally or in part, for non-commercial purposes provided that ECHA is acknowledged as the source: "Source: European Chemicals Agency, http://echa.europa.eu/". Such acknowledgement must be included in each copy of the material. ECHA permits and encourages organisations and individuals to create links to the ECHA website under the following cumulative conditions: Links can only be made to webpages that provide a link to the Legal Notice page. | |
Retrosynthesis Analysis
AI-Powered Synthesis Planning: Our tool employs the Template_relevance Pistachio, Template_relevance Bkms_metabolic, Template_relevance Pistachio_ringbreaker, Template_relevance Reaxys, Template_relevance Reaxys_biocatalysis model, leveraging a vast database of chemical reactions to predict feasible synthetic routes.
One-Step Synthesis Focus: Specifically designed for one-step synthesis, it provides concise and direct routes for your target compounds, streamlining the synthesis process.
Accurate Predictions: Utilizing the extensive PISTACHIO, BKMS_METABOLIC, PISTACHIO_RINGBREAKER, REAXYS, REAXYS_BIOCATALYSIS database, our tool offers high-accuracy predictions, reflecting the latest in chemical research and data.
Strategy Settings
Precursor scoring | Relevance Heuristic |
---|---|
Min. plausibility | 0.01 |
Model | Template_relevance |
Template Set | Pistachio/Bkms_metabolic/Pistachio_ringbreaker/Reaxys/Reaxys_biocatalysis |
Top-N result to add to graph | 6 |
Feasible Synthetic Routes
Q1: What is the molecular formula and weight of diphenylphosphinamide?
A1: The molecular formula of this compound is C12H12NOP, and its molecular weight is 217.21 g/mol. []
Q2: What spectroscopic data is available for this compound?
A2: Researchers commonly characterize this compound using techniques such as 1H NMR, 13C NMR, 31P NMR, and IR spectroscopy. Crystallographic data from X-ray diffraction studies are also frequently reported, providing insights into the compound's solid-state structure. [, , , ]
Q3: Is this compound air and moisture stable?
A3: Yes, this compound is generally considered air and moisture stable, making it convenient to handle and store. []
Q4: How is this compound used in organic synthesis?
A4: this compound is a valuable reagent for synthesizing a variety of compounds. It serves as a precursor for air-stable imines through the Kresze reaction. [, ] Additionally, it acts as a nitrogen nucleophile in reactions with aldehydes and alkynes, leading to the formation of N-diphenylphosphinoylimines and N-aryl-1,2-dihydropyridines, respectively. [, ]
Q5: What is the role of this compound in aza-Baylis–Hillman reactions?
A5: this compound participates in aza-Baylis–Hillman reactions with activated olefins, such as methyl vinyl ketone and methyl acrylate, in the presence of Lewis bases. This reaction provides access to a diverse range of multifunctional systems, including those with potential antitumor properties. [, ]
Q6: Can this compound be used in asymmetric synthesis?
A6: Yes, chiral phosphoramide ligands derived from this compound have demonstrated effectiveness in asymmetric synthesis. For example, N-((1R,2R)-2-(isopropylamino)cyclohexyl)-P,P-diphenylphosphinic amide (9c) catalyzes the addition of arylalkyl zincs to aromatic aldehydes, yielding chiral diarylmethanol compounds with high enantioselectivity (up to 94% ee). []
Q7: Have computational methods been used to study this compound?
A7: Yes, Density Functional Theory (DFT) calculations have been employed to investigate the mechanism of anionic dearomatizing reactions involving this compound derivatives. These studies provided insights into the reaction pathway and the influence of coordinating co-solvents. []
Q8: How do structural modifications of this compound affect its reactivity?
A8: Introducing substituents on the phenyl rings or modifying the nitrogen substituents can significantly influence the reactivity and coordination behavior of this compound. For example, ortho-lithiation allows for the introduction of various functional groups at the ortho position. [, ] The choice of N-substituents impacts the steric environment around the phosphorus center, influencing its coordination chemistry and catalytic activity. [, , ]
Q9: What is the significance of this compound in lanthanide coordination chemistry?
A9: this compound acts as a ligand in lanthanide complexes, coordinating to the metal center through its oxygen atom. These complexes have been extensively studied due to their interesting photophysical properties and potential applications as luminescent materials. [, , , , ]
Q10: How does the structure of this compound affect its coordination to lanthanide ions?
A10: The steric bulk of the phenyl groups and the nitrogen substituents on this compound can influence the coordination geometry and stability of the resulting lanthanide complexes. Researchers have investigated the impact of different substituents on the spectroscopic properties and structures of these complexes. [, , ]
Q11: Is there information available on the environmental impact of this compound?
A11: While specific data on the environmental impact of this compound might be limited in the provided research, it's crucial to consider the potential effects of organophosphorus compounds on the environment. Assessing factors like biodegradability and ecotoxicological effects is essential for responsible use and disposal. []
Disclaimer and Information on In-Vitro Research Products
Please be aware that all articles and product information presented on BenchChem are intended solely for informational purposes. The products available for purchase on BenchChem are specifically designed for in-vitro studies, which are conducted outside of living organisms. In-vitro studies, derived from the Latin term "in glass," involve experiments performed in controlled laboratory settings using cells or tissues. It is important to note that these products are not categorized as medicines or drugs, and they have not received approval from the FDA for the prevention, treatment, or cure of any medical condition, ailment, or disease. We must emphasize that any form of bodily introduction of these products into humans or animals is strictly prohibited by law. It is essential to adhere to these guidelines to ensure compliance with legal and ethical standards in research and experimentation.