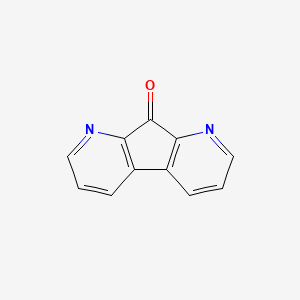
1,8-Diazafluoren-9-one
Overview
Description
1,8-Diazafluoren-9-one is an aromatic ketone first synthesized in 1950. It is known for its application in forensic science, particularly for detecting latent fingerprints on porous surfaces. The compound reacts with amino acids present in fingerprints to form highly fluorescent derivatives, making the fingerprints glow under blue-green light .
Mechanism of Action
Target of Action
The primary target of 1,8-Diazafluoren-9-one (DFO) is the amino acids present in latent fingerprints . These amino acids are left behind when fingers touch a surface, and they serve as the key interaction point for DFO .
Mode of Action
DFO interacts with its targets by forming highly fluorescent derivatives with the amino acids . This reaction is triggered when DFO comes into contact with the amino acids present in the fingerprint residue . The fluorescence of these derivatives is what allows the previously invisible fingerprints to be detected .
Biochemical Pathways
The biochemical pathway involved in the action of DFO is relatively straightforward. When DFO comes into contact with the amino acids present in fingerprint residue, it reacts to form fluorescent derivatives
Result of Action
The result of DFO’s action is the formation of fluorescent derivatives when it reacts with the amino acids present in fingerprint residue . These derivatives fluoresce under specific light conditions, allowing for the detection of previously invisible fingerprints . The fluorescence is typically observed when the derivatives are illuminated with light at an excitation wavelength of approximately 470 nm, resulting in emission at approximately 570 nm .
Action Environment
The action of DFO can be influenced by various environmental factors. For instance, the type of surface on which the fingerprints are located can affect the efficacy of DFO. It is most effective on porous surfaces . Additionally, the fluorescence of the DFO-amino acid derivatives can be affected by the lighting conditions, specifically the wavelength of light used for excitation . Furthermore, the concentration of DFO and the solvent used can also influence the fluorescence intensity .
Biochemical Analysis
Biochemical Properties
1,8-Diazafluoren-9-one plays a significant role in biochemical reactions, particularly in forensic applications. It interacts with amino acids such as L-alanine to form fluorescent derivatives. This interaction involves the formation of a hemiketal intermediate, which is the reactive species in the reaction . The compound’s ability to form fluorescent derivatives with amino acids is due to its unique chemical structure, which includes nitrogen and oxygen heteroatoms in a ring structure .
Cellular Effects
The effects of this compound on various types of cells and cellular processes are primarily related to its fluorescent properties. When used in forensic applications, the compound does not significantly impact cell function, signaling pathways, gene expression, or cellular metabolism. Its interaction with amino acids in fingerprint residues highlights its potential for biochemical applications beyond forensics .
Molecular Mechanism
At the molecular level, this compound exerts its effects through binding interactions with amino acids. The compound reacts with amino acids to form fluorescent derivatives, which can be detected using fluorescence spectroscopy. This reaction involves the formation of a hemiketal intermediate, which is the reactive species that binds to the amino acids . The fluorescence properties of the derivatives are due to the unique structure of this compound, which allows for efficient energy transfer and emission of light .
Temporal Effects in Laboratory Settings
In laboratory settings, the effects of this compound can change over time due to its stability and degradation. The compound is stable under standard laboratory conditions, but its fluorescent properties can degrade over time, especially when exposed to light and air. Long-term studies have shown that the compound remains effective for detecting fingerprints for extended periods, but its fluorescence intensity may decrease over time .
Metabolic Pathways
The metabolic pathways of this compound involve its interaction with amino acids to form fluorescent derivatives. The compound does not undergo significant metabolic changes in the body, as it is primarily used for external applications in forensic science. Its interaction with amino acids highlights its potential for use in biochemical assays and diagnostic applications .
Transport and Distribution
This compound is transported and distributed within cells and tissues primarily through passive diffusion. The compound’s small size and lipophilic nature allow it to easily penetrate cell membranes and interact with intracellular amino acids. Its distribution within tissues is influenced by its affinity for amino acids and other biomolecules .
Subcellular Localization
The subcellular localization of this compound is primarily in the cytoplasm, where it interacts with amino acids to form fluorescent derivatives. The compound does not have specific targeting signals or post-translational modifications that direct it to specific compartments or organelles. Its activity and function are mainly determined by its ability to form fluorescent derivatives with amino acids in the cytoplasm .
Preparation Methods
Synthetic Routes and Reaction Conditions
1,8-Diazafluoren-9-one can be synthesized through various methods. One common synthetic route involves the reaction of 2,2’-bipyridine with phosgene, followed by cyclization and oxidation steps. The reaction conditions typically involve the use of solvents such as methanol and ethyl acetate, with glacial acetic acid as a catalyst .
Industrial Production Methods
In industrial settings, the preparation of this compound involves similar synthetic routes but on a larger scale. The process includes the preparation of a stock solution by dissolving the compound in methanol and ethyl acetate, followed by dilution with petroleum ether to form a working solution. This working solution is then used for various applications, including forensic analysis .
Chemical Reactions Analysis
Types of Reactions
1,8-Diazafluoren-9-one undergoes several types of chemical reactions, including:
Oxidation: The compound can be oxidized to form various derivatives.
Reduction: Reduction reactions can convert it into different reduced forms.
Substitution: It can undergo substitution reactions with various reagents.
Common Reagents and Conditions
Oxidation: Common oxidizing agents include potassium permanganate and hydrogen peroxide.
Reduction: Reducing agents such as sodium borohydride are often used.
Substitution: Substitution reactions typically involve reagents like halogens and nucleophiles under controlled conditions.
Major Products Formed
The major products formed from these reactions depend on the specific reagents and conditions used. For example, oxidation can lead to the formation of carboxylic acids, while reduction can yield alcohols or amines .
Scientific Research Applications
1,8-Diazafluoren-9-one has a wide range of scientific research applications:
Forensic Science: It is extensively used for detecting latent fingerprints on porous surfaces by forming fluorescent derivatives with amino acids.
Optical Applications: The compound is used in the development of luminescent materials for optical applications, such as thin films and composite materials.
Biological Research: It is employed in the detection of α-amino acids, which are potential markers for various diseases.
Industrial Applications: The compound is used in the production of various chemical products and materials due to its unique chemical properties.
Comparison with Similar Compounds
Similar Compounds
Ninhydrin: Another compound used for detecting fingerprints, but it forms a purple product instead of a fluorescent one.
2,4-Dinitrophenylhydrazine: Used for detecting carbonyl compounds, but not as effective for fingerprint detection.
Uniqueness
1,8-Diazafluoren-9-one is unique due to its ability to form highly fluorescent derivatives with amino acids, making it particularly useful in forensic science for detecting latent fingerprints. Its fluorescence properties and the ability to form stable derivatives set it apart from other similar compounds .
Properties
IUPAC Name |
6,10-diazatricyclo[7.4.0.02,7]trideca-1(9),2(7),3,5,10,12-hexaen-8-one | |
---|---|---|
Source | PubChem | |
URL | https://pubchem.ncbi.nlm.nih.gov | |
Description | Data deposited in or computed by PubChem | |
InChI |
InChI=1S/C11H6N2O/c14-11-9-7(3-1-5-12-9)8-4-2-6-13-10(8)11/h1-6H | |
Source | PubChem | |
URL | https://pubchem.ncbi.nlm.nih.gov | |
Description | Data deposited in or computed by PubChem | |
InChI Key |
FOSUVSBKUIWVKI-UHFFFAOYSA-N | |
Source | PubChem | |
URL | https://pubchem.ncbi.nlm.nih.gov | |
Description | Data deposited in or computed by PubChem | |
Canonical SMILES |
C1=CC2=C(C(=O)C3=C2C=CC=N3)N=C1 | |
Source | PubChem | |
URL | https://pubchem.ncbi.nlm.nih.gov | |
Description | Data deposited in or computed by PubChem | |
Molecular Formula |
C11H6N2O | |
Source | PubChem | |
URL | https://pubchem.ncbi.nlm.nih.gov | |
Description | Data deposited in or computed by PubChem | |
DSSTOX Substance ID |
DTXSID10352406 | |
Record name | 9H-Cyclopenta[1,2-b:4,3-b']dipyridin-9-one | |
Source | EPA DSSTox | |
URL | https://comptox.epa.gov/dashboard/DTXSID10352406 | |
Description | DSSTox provides a high quality public chemistry resource for supporting improved predictive toxicology. | |
Molecular Weight |
182.18 g/mol | |
Source | PubChem | |
URL | https://pubchem.ncbi.nlm.nih.gov | |
Description | Data deposited in or computed by PubChem | |
CAS No. |
54078-29-4 | |
Record name | 9H-Cyclopenta[1,2-b:4,3-b′]dipyridin-9-one | |
Source | CAS Common Chemistry | |
URL | https://commonchemistry.cas.org/detail?cas_rn=54078-29-4 | |
Description | CAS Common Chemistry is an open community resource for accessing chemical information. Nearly 500,000 chemical substances from CAS REGISTRY cover areas of community interest, including common and frequently regulated chemicals, and those relevant to high school and undergraduate chemistry classes. This chemical information, curated by our expert scientists, is provided in alignment with our mission as a division of the American Chemical Society. | |
Explanation | The data from CAS Common Chemistry is provided under a CC-BY-NC 4.0 license, unless otherwise stated. | |
Record name | 1,8-Diazafluoren-9-one | |
Source | ChemIDplus | |
URL | https://pubchem.ncbi.nlm.nih.gov/substance/?source=chemidplus&sourceid=0054078294 | |
Description | ChemIDplus is a free, web search system that provides access to the structure and nomenclature authority files used for the identification of chemical substances cited in National Library of Medicine (NLM) databases, including the TOXNET system. | |
Record name | 9H-Cyclopenta[1,2-b:4,3-b']dipyridin-9-one | |
Source | EPA DSSTox | |
URL | https://comptox.epa.gov/dashboard/DTXSID10352406 | |
Description | DSSTox provides a high quality public chemistry resource for supporting improved predictive toxicology. | |
Record name | 1,8-DIAZAFLUOREN-9-ONE | |
Source | FDA Global Substance Registration System (GSRS) | |
URL | https://gsrs.ncats.nih.gov/ginas/app/beta/substances/V3B9KD3X5K | |
Description | The FDA Global Substance Registration System (GSRS) enables the efficient and accurate exchange of information on what substances are in regulated products. Instead of relying on names, which vary across regulatory domains, countries, and regions, the GSRS knowledge base makes it possible for substances to be defined by standardized, scientific descriptions. | |
Explanation | Unless otherwise noted, the contents of the FDA website (www.fda.gov), both text and graphics, are not copyrighted. They are in the public domain and may be republished, reprinted and otherwise used freely by anyone without the need to obtain permission from FDA. Credit to the U.S. Food and Drug Administration as the source is appreciated but not required. | |
Synthesis routes and methods I
Procedure details
Synthesis routes and methods II
Procedure details
Retrosynthesis Analysis
AI-Powered Synthesis Planning: Our tool employs the Template_relevance Pistachio, Template_relevance Bkms_metabolic, Template_relevance Pistachio_ringbreaker, Template_relevance Reaxys, Template_relevance Reaxys_biocatalysis model, leveraging a vast database of chemical reactions to predict feasible synthetic routes.
One-Step Synthesis Focus: Specifically designed for one-step synthesis, it provides concise and direct routes for your target compounds, streamlining the synthesis process.
Accurate Predictions: Utilizing the extensive PISTACHIO, BKMS_METABOLIC, PISTACHIO_RINGBREAKER, REAXYS, REAXYS_BIOCATALYSIS database, our tool offers high-accuracy predictions, reflecting the latest in chemical research and data.
Strategy Settings
Precursor scoring | Relevance Heuristic |
---|---|
Min. plausibility | 0.01 |
Model | Template_relevance |
Template Set | Pistachio/Bkms_metabolic/Pistachio_ringbreaker/Reaxys/Reaxys_biocatalysis |
Top-N result to add to graph | 6 |
Feasible Synthetic Routes
Disclaimer and Information on In-Vitro Research Products
Please be aware that all articles and product information presented on BenchChem are intended solely for informational purposes. The products available for purchase on BenchChem are specifically designed for in-vitro studies, which are conducted outside of living organisms. In-vitro studies, derived from the Latin term "in glass," involve experiments performed in controlled laboratory settings using cells or tissues. It is important to note that these products are not categorized as medicines or drugs, and they have not received approval from the FDA for the prevention, treatment, or cure of any medical condition, ailment, or disease. We must emphasize that any form of bodily introduction of these products into humans or animals is strictly prohibited by law. It is essential to adhere to these guidelines to ensure compliance with legal and ethical standards in research and experimentation.