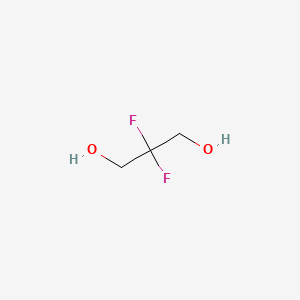
2,2-Difluoropropane-1,3-diol
Overview
Description
2,2-Difluoropropane-1,3-diol is an organic compound with the molecular formula C3H6F2O2. It is a colorless liquid with a unique structure characterized by the presence of two fluorine atoms attached to the second carbon of a propane-1,3-diol backbone. This compound is known for its versatility and is used in various fields, including pharmaceuticals, agrochemicals, and polymer synthesis .
Biochemical Analysis
Biochemical Properties
2,2-Difluoropropane-1,3-diol plays a significant role in biochemical reactions due to its unique structure. The compound interacts with various enzymes and proteins, influencing their activity. For instance, it is used as an intermediate in the synthesis of pharmaceutical compounds such as anti-cancer agents and antibiotics. The fluorine atoms in this compound enhance its binding affinity to certain enzymes, thereby modulating their activity. Additionally, the compound can interact with proteins involved in cell signaling pathways, affecting their function and stability .
Cellular Effects
The effects of this compound on various types of cells and cellular processes are profound. The compound influences cell function by modulating cell signaling pathways, gene expression, and cellular metabolism. For example, this compound can alter the expression of genes involved in metabolic pathways, leading to changes in cellular metabolism. It also affects cell signaling pathways by interacting with key proteins, thereby influencing cellular responses to external stimuli .
Molecular Mechanism
At the molecular level, this compound exerts its effects through various mechanisms. The compound can bind to specific biomolecules, such as enzymes and proteins, altering their activity. For instance, it can inhibit or activate enzymes involved in metabolic pathways, leading to changes in metabolic flux. Additionally, this compound can influence gene expression by interacting with transcription factors and other regulatory proteins, thereby modulating the transcription of specific genes .
Temporal Effects in Laboratory Settings
In laboratory settings, the effects of this compound can change over time. The compound’s stability and degradation are important factors that influence its long-term effects on cellular function. Studies have shown that this compound is relatively stable under ambient conditions, but it can degrade over time, leading to changes in its biological activity. Long-term exposure to the compound can result in alterations in cellular function, including changes in gene expression and metabolic pathways .
Dosage Effects in Animal Models
The effects of this compound vary with different dosages in animal models. At low doses, the compound may have minimal effects on cellular function, while at higher doses, it can cause significant changes in cell signaling pathways and gene expression. Threshold effects have been observed, where a certain dosage is required to elicit a biological response. Additionally, high doses of this compound can lead to toxic or adverse effects, including cellular damage and disruption of metabolic processes .
Metabolic Pathways
This compound is involved in various metabolic pathways, interacting with enzymes and cofactors. The compound can be metabolized by enzymes such as dehydrogenases and reductases, leading to the formation of metabolites that can further participate in biochemical reactions. The presence of fluorine atoms in this compound can influence its metabolic flux, affecting the levels of metabolites in the cell .
Transport and Distribution
The transport and distribution of this compound within cells and tissues are crucial for its biological activity. The compound can be transported across cell membranes by specific transporters and binding proteins. Once inside the cell, this compound can accumulate in certain cellular compartments, influencing its localization and activity. The distribution of the compound within tissues can also affect its overall biological effects .
Subcellular Localization
The subcellular localization of this compound plays a key role in its activity and function. The compound can be directed to specific compartments or organelles within the cell through targeting signals or post-translational modifications. For example, this compound may localize to the mitochondria, where it can influence mitochondrial function and energy metabolism. The localization of the compound within the cell can also affect its interactions with other biomolecules and its overall biological activity .
Preparation Methods
Synthetic Routes and Reaction Conditions: 2,2-Difluoropropane-1,3-diol can be synthesized through several methods:
Hydrolysis of 2,2-difluoro-1,3-propanedithiol: This method involves the hydrolysis of 2,2-difluoro-1,3-propanedithiol using an aqueous solution of sodium hydroxide or potassium hydroxide, resulting in the formation of this compound.
Reaction of Hydrogen Fluoride with Propylene Glycol: Another common method involves the reaction of hydrogen fluoride with propylene glycol under controlled conditions.
Industrial Production Methods: In industrial settings, the production of this compound typically involves the large-scale reaction of hydrogen fluoride with propylene glycol. This method is favored due to its efficiency and the availability of raw materials .
Chemical Reactions Analysis
2,2-Difluoropropane-1,3-diol undergoes various chemical reactions, including:
Oxidation: The compound can be oxidized to form corresponding carbonyl compounds using oxidizing agents such as potassium permanganate or chromium trioxide.
Reduction: Reduction reactions can convert this compound into its corresponding alcohols using reducing agents like lithium aluminum hydride.
Substitution: The fluorine atoms in the compound can be substituted with other functional groups using nucleophilic substitution reactions.
Major Products Formed:
Oxidation Products: Carbonyl compounds such as aldehydes and ketones.
Reduction Products: Alcohols.
Substitution Products: Compounds with various functional groups replacing the fluorine atoms.
Scientific Research Applications
2,2-Difluoropropane-1,3-diol has several applications in scientific research:
Pharmaceuticals: It is used as an intermediate in the synthesis of various pharmaceutical compounds, including anti-cancer agents and antibiotics.
Agrochemicals: The compound is used in the synthesis of herbicides and insecticides.
Fluorinated Surfactants: this compound is used to produce fluorinated surfactants, which have unique properties such as oil and water repellency.
Polymer Synthesis: The compound serves as a monomer in the synthesis of fluorinated polymers used in coatings, adhesives, and membranes.
Mechanism of Action
The mechanism of action of 2,2-difluoropropane-1,3-diol is primarily related to its ability to participate in various chemical reactions due to the presence of fluorine atoms. These fluorine atoms impart unique properties such as hydrophobicity and thermal stability, making the compound effective in its applications. The molecular targets and pathways involved depend on the specific application, such as enzyme inhibition in pharmaceuticals or interaction with other chemicals in agrochemicals .
Comparison with Similar Compounds
2,2-Difluoropropane-1,3-diol can be compared with other similar compounds, such as:
2,2-Difluoroethanol: Similar in structure but with one less carbon atom, making it less versatile in certain applications.
2,2-Difluorobutane-1,4-diol: Contains an additional carbon atom, which can affect its reactivity and applications.
2,2-Difluoropropane-1,2-diol: Differing in the position of the hydroxyl groups, which can influence its chemical behavior.
The uniqueness of this compound lies in its specific structure, which provides a balance of reactivity and stability, making it suitable for a wide range of applications .
Biological Activity
2,2-Difluoropropane-1,3-diol (DFPD) is a fluorinated organic compound with significant implications in biochemical and pharmaceutical research. Its unique structure, characterized by the presence of two fluorine atoms, enhances its biological activity and interaction with various biomolecules. This article delves into the biological activity of DFPD, exploring its biochemical properties, cellular effects, mechanisms of action, and applications in scientific research.
DFPD exhibits a range of biochemical properties that make it a valuable compound in medicinal chemistry. The fluorine atoms contribute to its increased lipophilicity and metabolic stability compared to non-fluorinated analogs. This compound serves as an intermediate in synthesizing various pharmaceuticals, including anti-cancer agents and antibiotics .
Property | Value/Description |
---|---|
Molecular Formula | C₃H₆F₂O₂ |
Molecular Weight | 110.08 g/mol |
Solubility | Soluble in organic solvents |
Stability | Relatively stable under ambient conditions but degrades over time |
Cellular Effects
DFPD influences cellular functions by modulating various signaling pathways and gene expressions. Research indicates that this compound can alter metabolic pathways by interacting with key enzymes and proteins involved in cellular metabolism. For instance, it has been observed to affect the expression of genes associated with metabolic regulation.
Key Findings:
- Gene Expression : DFPD can upregulate or downregulate genes involved in metabolic processes.
- Cell Signaling : It interacts with proteins that are crucial for cell signaling, impacting cellular responses to stimuli.
The biological activity of DFPD primarily stems from its ability to bind to specific biomolecules like enzymes and proteins. The presence of fluorine enhances the binding affinity, thereby modulating enzyme activities:
- Enzyme Interaction : DFPD can inhibit or activate enzymes that regulate metabolic pathways.
- Transcription Factors : It may influence gene transcription by interacting with regulatory proteins.
Temporal and Dosage Effects
The effects of DFPD can vary significantly over time and dosage levels:
- Temporal Stability : Although stable initially, prolonged exposure can lead to degradation and altered biological activity.
- Dosage Response : Low doses may have minimal effects on cellular functions, while higher doses can lead to significant changes in signaling pathways and potential toxicity.
Table 2: Dosage Effects Observed in Animal Models
Dose Level (mg/kg) | Observed Effects |
---|---|
Low (1-10) | Minimal impact on cellular functions |
Moderate (10-50) | Altered gene expression |
High (>50) | Significant toxicity and cellular damage |
Applications in Scientific Research
DFPD has several applications across different fields:
- Pharmaceuticals : Used as an intermediate for synthesizing anti-cancer drugs and antibiotics.
- Agrochemicals : Involved in developing herbicides and insecticides due to its reactive nature .
- Fluorinated Polymers : Acts as a monomer for producing fluorinated polymers with unique properties suitable for coatings and adhesives.
Case Studies
Several studies have highlighted the efficacy of DFPD in various applications:
- Synthesis of Anti-Cancer Agents : Research demonstrated that DFPD derivatives showed enhanced cytotoxicity against cancer cell lines compared to non-fluorinated counterparts.
- Gene Regulation Studies : Experiments indicated that DFPD could modulate the expression of specific genes involved in apoptosis, suggesting potential therapeutic applications in cancer treatment .
Properties
IUPAC Name |
2,2-difluoropropane-1,3-diol | |
---|---|---|
Source | PubChem | |
URL | https://pubchem.ncbi.nlm.nih.gov | |
Description | Data deposited in or computed by PubChem | |
InChI |
InChI=1S/C3H6F2O2/c4-3(5,1-6)2-7/h6-7H,1-2H2 | |
Source | PubChem | |
URL | https://pubchem.ncbi.nlm.nih.gov | |
Description | Data deposited in or computed by PubChem | |
InChI Key |
AMCKYDINHDOOCB-UHFFFAOYSA-N | |
Source | PubChem | |
URL | https://pubchem.ncbi.nlm.nih.gov | |
Description | Data deposited in or computed by PubChem | |
Canonical SMILES |
C(C(CO)(F)F)O | |
Source | PubChem | |
URL | https://pubchem.ncbi.nlm.nih.gov | |
Description | Data deposited in or computed by PubChem | |
Molecular Formula |
C3H6F2O2 | |
Source | PubChem | |
URL | https://pubchem.ncbi.nlm.nih.gov | |
Description | Data deposited in or computed by PubChem | |
DSSTOX Substance ID |
DTXSID20195487 | |
Record name | 1,3-Propanediol, 2,2-difluoro- | |
Source | EPA DSSTox | |
URL | https://comptox.epa.gov/dashboard/DTXSID20195487 | |
Description | DSSTox provides a high quality public chemistry resource for supporting improved predictive toxicology. | |
Molecular Weight |
112.08 g/mol | |
Source | PubChem | |
URL | https://pubchem.ncbi.nlm.nih.gov | |
Description | Data deposited in or computed by PubChem | |
CAS No. |
428-63-7 | |
Record name | 1,3-Propanediol, 2,2-difluoro- | |
Source | ChemIDplus | |
URL | https://pubchem.ncbi.nlm.nih.gov/substance/?source=chemidplus&sourceid=0000428637 | |
Description | ChemIDplus is a free, web search system that provides access to the structure and nomenclature authority files used for the identification of chemical substances cited in National Library of Medicine (NLM) databases, including the TOXNET system. | |
Record name | 428-63-7 | |
Source | DTP/NCI | |
URL | https://dtp.cancer.gov/dtpstandard/servlet/dwindex?searchtype=NSC&outputformat=html&searchlist=32744 | |
Description | The NCI Development Therapeutics Program (DTP) provides services and resources to the academic and private-sector research communities worldwide to facilitate the discovery and development of new cancer therapeutic agents. | |
Explanation | Unless otherwise indicated, all text within NCI products is free of copyright and may be reused without our permission. Credit the National Cancer Institute as the source. | |
Record name | 1,3-Propanediol, 2,2-difluoro- | |
Source | EPA DSSTox | |
URL | https://comptox.epa.gov/dashboard/DTXSID20195487 | |
Description | DSSTox provides a high quality public chemistry resource for supporting improved predictive toxicology. | |
Record name | 2,2-Difluoropropane-1,3-diol | |
Source | European Chemicals Agency (ECHA) | |
URL | https://echa.europa.eu/information-on-chemicals | |
Description | The European Chemicals Agency (ECHA) is an agency of the European Union which is the driving force among regulatory authorities in implementing the EU's groundbreaking chemicals legislation for the benefit of human health and the environment as well as for innovation and competitiveness. | |
Explanation | Use of the information, documents and data from the ECHA website is subject to the terms and conditions of this Legal Notice, and subject to other binding limitations provided for under applicable law, the information, documents and data made available on the ECHA website may be reproduced, distributed and/or used, totally or in part, for non-commercial purposes provided that ECHA is acknowledged as the source: "Source: European Chemicals Agency, http://echa.europa.eu/". Such acknowledgement must be included in each copy of the material. ECHA permits and encourages organisations and individuals to create links to the ECHA website under the following cumulative conditions: Links can only be made to webpages that provide a link to the Legal Notice page. | |
Retrosynthesis Analysis
AI-Powered Synthesis Planning: Our tool employs the Template_relevance Pistachio, Template_relevance Bkms_metabolic, Template_relevance Pistachio_ringbreaker, Template_relevance Reaxys, Template_relevance Reaxys_biocatalysis model, leveraging a vast database of chemical reactions to predict feasible synthetic routes.
One-Step Synthesis Focus: Specifically designed for one-step synthesis, it provides concise and direct routes for your target compounds, streamlining the synthesis process.
Accurate Predictions: Utilizing the extensive PISTACHIO, BKMS_METABOLIC, PISTACHIO_RINGBREAKER, REAXYS, REAXYS_BIOCATALYSIS database, our tool offers high-accuracy predictions, reflecting the latest in chemical research and data.
Strategy Settings
Precursor scoring | Relevance Heuristic |
---|---|
Min. plausibility | 0.01 |
Model | Template_relevance |
Template Set | Pistachio/Bkms_metabolic/Pistachio_ringbreaker/Reaxys/Reaxys_biocatalysis |
Top-N result to add to graph | 6 |
Feasible Synthetic Routes
Q1: What are the key synthetic routes for producing 2,2-Difluoropropane-1,3-diol?
A1: A recent study highlights the use of Difluoromethyl Phenyl Sulfone as a versatile reagent for the synthesis of anti-2,2-Difluoropropane-1,3-diols. [, ] This method leverages the reagent's ability to act as a selective difluoromethylene dianion equivalent, enabling a one-pot stereoselective synthesis. [] Another approach involves the reaction of difluoromethyl aryl ketones with aryl aldehydes in the presence of potassium tert-butoxide. This method, conducted in anhydrous dimethylformamide (DMF), facilitates the creation of both symmetrical and unsymmetrical 2,2-Difluoropropane-1,3-diols with moderate to good yields. []
Q2: What are the advantages of using Difluoromethyl Phenyl Sulfone in the synthesis of this compound?
A2: Difluoromethyl Phenyl Sulfone exhibits high selectivity as a difluoromethylene dianion equivalent, leading to the efficient and stereoselective formation of anti-2,2-Difluoropropane-1,3-diols. [] This selectivity simplifies the synthesis process and enhances the yield of the desired diastereomer. Furthermore, the one-pot reaction design using this reagent contributes to the method's overall efficiency and practicality. []
Q3: What are the potential applications of 2,2-Difluoropropane-1,3-diols in medicinal chemistry?
A3: While the provided research focuses primarily on the synthesis of 2,2-Difluoropropane-1,3-diols, their unique structure, particularly the presence of fluorine atoms, suggests potential applications in medicinal chemistry. The incorporation of fluorine into organic molecules can significantly impact their metabolic stability, lipophilicity, and binding affinity to target proteins. [] Therefore, 2,2-Difluoropropane-1,3-diols could serve as valuable building blocks for developing novel pharmaceuticals or agrochemicals with improved properties.
- Direct Aldol–Reduction Process using Difluoromethyl Aryl Ketones and Aryl Aldehydes in the Presence of Potassium tert-Butoxide: One-Pot Efficient Stereoselective Synthesis of Symmetrical and Unsymmetrical anti-2,2-Difluoropropane-1,3-diols:
- Difluoromethyl Phenyl Sulfone as a Selective Difluoromethylene Dianion Equivalent: One‐Pot Stereoselective Synthesis of anti‐2,2‐Difluoropropane‐1,3‐diols:
- Difluoromethyl phenyl sulfone as a selective difluoromethylene dianion equivalent: one-pot stereoselective synthesis of anti-2,2-difluoropropane-1,3-diols:
- Difluoromethyl Phenyl Sulfone:
Disclaimer and Information on In-Vitro Research Products
Please be aware that all articles and product information presented on BenchChem are intended solely for informational purposes. The products available for purchase on BenchChem are specifically designed for in-vitro studies, which are conducted outside of living organisms. In-vitro studies, derived from the Latin term "in glass," involve experiments performed in controlled laboratory settings using cells or tissues. It is important to note that these products are not categorized as medicines or drugs, and they have not received approval from the FDA for the prevention, treatment, or cure of any medical condition, ailment, or disease. We must emphasize that any form of bodily introduction of these products into humans or animals is strictly prohibited by law. It is essential to adhere to these guidelines to ensure compliance with legal and ethical standards in research and experimentation.